My notes from reading the "Guyton and Hall Textbook of Medical Physiology" textbook so that I can better understand how the body works.
read moreFundamentally, you've just got a bunch of cells in the body, and they're all interacting with the fluid in the blood stream, which diffuses into the cell. Now the blood stream needs to have the right balance of ions, nutrients, etc to keep all the cells working properly, and also it's important to note that every single cell is getting the same stuff, because it's just getting it from the uniform contents of the bloodstream.
Your body is always trying to maintain homeostasis. You eat a ton of salt, it has mechanisms for making sure your blood sodium concentration isn't going to go through the roof. A disease is really anything that disrupts homeostasis. Your body will likely try to compensate for the disease by changing something so you don't die immediately, but left unchecked, the compensation may lead to longer term consequences.
The kidneys are specifically filtering most of the byproducts of the cells except carbon dioxide which is done by the lungs, such as: urea, uric acid, excess water, excess ions (hydrogen, sodium, potassium, phosphate).
The liver and pancreas regulate the concentration of glucose.
Nervous system is just the brain and the spinal cord, mostly, pretty clear what it does. An important part of the nervous system though is the autonomic system, which is completely subconscious and pumps the heart, moves the gastrointestinal tract, etc. Now similarly, to control all of the organs and their functions on a metabolic level, whereas the nervous system controls the functions on a physical level (valves opening, etc.), there's the hormone system. For instance, thyroid hormone increases the rate of chemical reactions in the cells, basically speeding things up or down (this is actually the big determiner of your basal metabolic rate). Insulin does glucose metabolism, adrenocortical hormones affect sodium and potassium ions.
Then you've got the immune system, which is the protector. Various components are the white blood cells, thymus, lymph nodes, etc. They both identify foreign cells to destroy and actually destroy them or neutralize them (sometimes capturing them for destruction, other times producing proteins called anti-bodies that attach and neutralize the invader.
And there's the skin, the Integumentary system. Also to protect, similar cot the immune system.
You also have the baroreceptor system, which are in the carotid arteries of the neck as well as the thorax (chest), which are activated by stretching of the arterial walls. They stretch too much (high BP), they send signal to the medulla (that really base part of brain), which reduces pumping activity, thus helping to reduce blood pressure.
Here are the normal ranges for oxygen, sodium, potassium, calcium, chloride, bicarbonate, glucose, body temp, and acid-base:
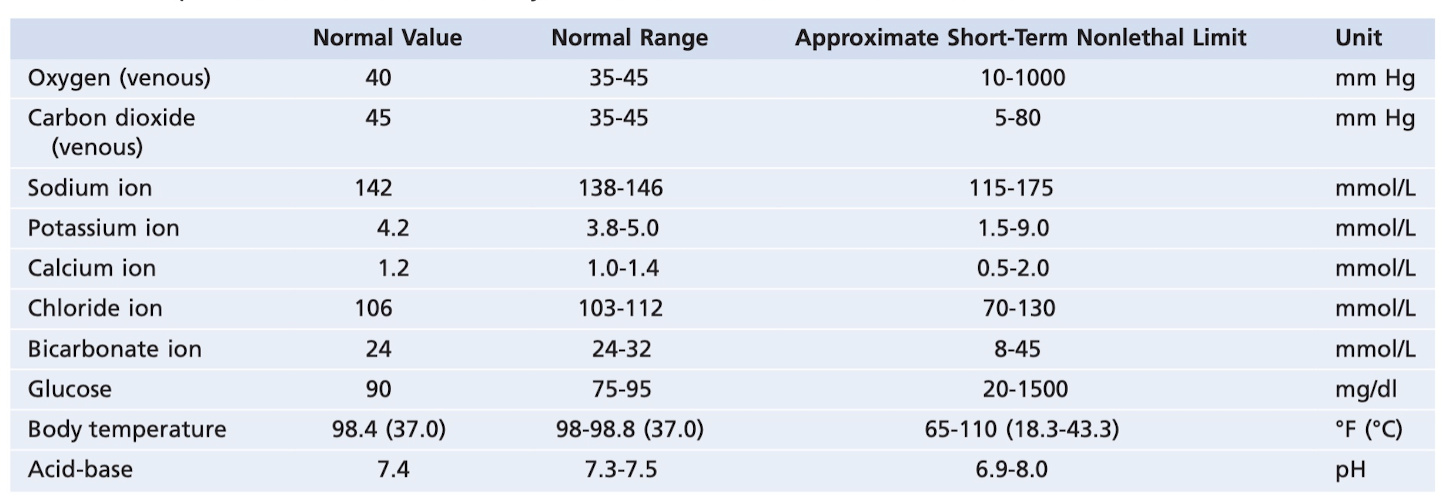
Consequences of being outside these limits: temp > 11F, spiral of increased cellular metabolism causing cell death. Potassium ion too low, paralyzed (nerve signals fail), calcium ion too low, constant contraction of muscles (called tetanic contractions, as in tetanus), glucose below one-half normal, extreme mental irritability and sometimes even convulsions. All of the mechanisms of control to maintain this homeostasis are negative feedback systems (thing goes up, something happens to bring it down; positive feedback is thing goes up, other thing causes it to go up more).
Positive feedback loops (also called vicious cycles, as in thing goes up, causes thing to go up more) often lead to death. For instance, for exsanguination, if you lose 2 liters of blood, your heart isn't as able to pump effectively, leading to the blood vessels on the hearth themselves not getting enough blood, leading to it not pumping as effectively, etc. until eventually the heart stops after about 2 hours. If it's less than 2 liters, then the control mechanisms can compensate enough to keep you alive. That said, sometimes positive feedback is useful, like blood clots. Clotting of blood causes more clotting until the ruptured blood vessel is plugged. Another example of bad positive feedback loops is fibrillation (of the heart).
The coronary artery is so essential because it's what provides blood to the muscles of the heart. It goes, the heart goes. Heart attacks are most commonly caused by plague buildup (mix of cholesterol and other things), atherosclerosis). This is why cholesterol intake is so important. Note that this cannot be reversed, the plague buildup is essentially permanent. Eventually, the plague ruptures (sometimes because rapid increase in blood pressure, like during strained physical activity), unfortunately the body tries to cover this rupture with a blood clot, which ends up blocking the blood flow entirely. That's the most common cause of an acute heart attack. This is actually why some people will take aspirin daily, because it reduces clotting. It's only recommended if you have a history of heart attacks or are at high risk, etc. The downsides of taking it regularly of course are if you do start bleeding somewhere, it'll be harder for your body to stop it, and internal bleeding could be of particular concern.
Tetanus (also called lockjaw, since it often affects the jaw) is caused by a bacteria in nearly all soil. If it gets into the blood stream through a cut, it'll fuck with your CNS, presumably and cause your muscles to constantly contract, eventually leading to death. The reason nearly nobody gets it is because nearly everyone is vaccinated as a child. Adults need the re-up shot every 10 years.
The cell is the building block of everything in the body. Organs are just collections of certain types of cells, etc. Important ions that exist in the cell are potassium, magnesium, phosphate, sulfate, bicarbonate, and smaller quantities of sodium, chloride, and calcium. Cholesterol and phospholipids are important in the cell because they're not water soluble, so they can form the cell membrane (the boundaries) to keep things inside. The cell wall is basically just lipids (as stated above) and proteins which kind of cut through the membrane and are the conduit through which things can travel in and out of the cell. The lipid layer is perfect for the cell membrane, because ions, glucose, etc can't move freely, but fat-soluble substances like oxygen and carbon dioxide can, so diffusion can occur. The balance of what's inside vs outside the cell is interesting, so like glucose outside is 90 mg/dl but inside it's 0 to 20 mg / dl, there's plenty of sodium ions outside the cell, and there's plenty of potassium inside the cell.
Of all the cell stuff, one organelle (just a name for component of a cell) that actually is pretty interesting is the lysosome. They are the intracellular digestive system, which allows the cell to eat (break down into constituent parts) damaged cell structures, food particles (this would be the case of the villi in the small intestine, presumably), as well as unwanted invaders like bacteria. It does this using hydrolase (digestive) enzymes. A hydrolytic enzyme breaks shit down into parts by taking a water molecule, breaking off the hydrogen and attaching it to one part and taking the rest (called hydroxyl, just OH, rather than H2O) and attaching it to another part. The result is called "hydrolyzed". Easy examples: protein is hydrolyzed to form amino acids, glycogen is hydrolyzed to form glucose, and lips are hydrolyzed to form fatty acids and glycerol. Lysosomes come into play when you have damaged tissue or damaged cells, like from heat, cold, trauma, chemicals. When the damage occurs, the lysosomes rupture, and all of the hydrolase is released and just start to eat everything up. If the cell is damaged enough, the entire cell is digested (called autolysis). As for eating up bacteria, the lysosome has a couple of different ways of killing the bacteria: fucking its membrane, inhibiting growth with iron, and shooting acid at it to inactivate it from multiplying. Autophagy is another term, which means to eat oneself, and it refers to worn-out organelles getting eaten up by the lysosomes. In liver cells, the mitochondrion usually only lives 10 days before it's destroyed.
The Golgi apparatus does also have a rather interesting function, it can synthesize certain carbohydrates like hyaluronic acid and chondroitin sulfate (called Glycosaminoglycan, or GAGs). These things are in mucus, they're major components in the extracellular matrix (ECM) which is the mass that fills the space between cells (also, the protein Collagen is here, like in the skin. Collagen is actually the most abundant protein in the body), and they're part of cartilage and bone. Below is a great diagram of the secretion of the stuff that are being produced. To get a sense for the timing: he following example provides an idea of the timing of these processes: When a glandular cell is bathed in radioactive amino acids, newly formed radioactive protein molecules can be detected in the granular endoplasmic reticulum within 3 to 5 minutes. Within 20 minutes, newly formed proteins are already present in the Golgi apparatus, and within 1 to 2 hours, the proteins are secreted from the surface of the cell.
Interstitial (pronounced "inter-stish-al) fluid just refers to the fluid that sits between cells basically, outside of the circulation.
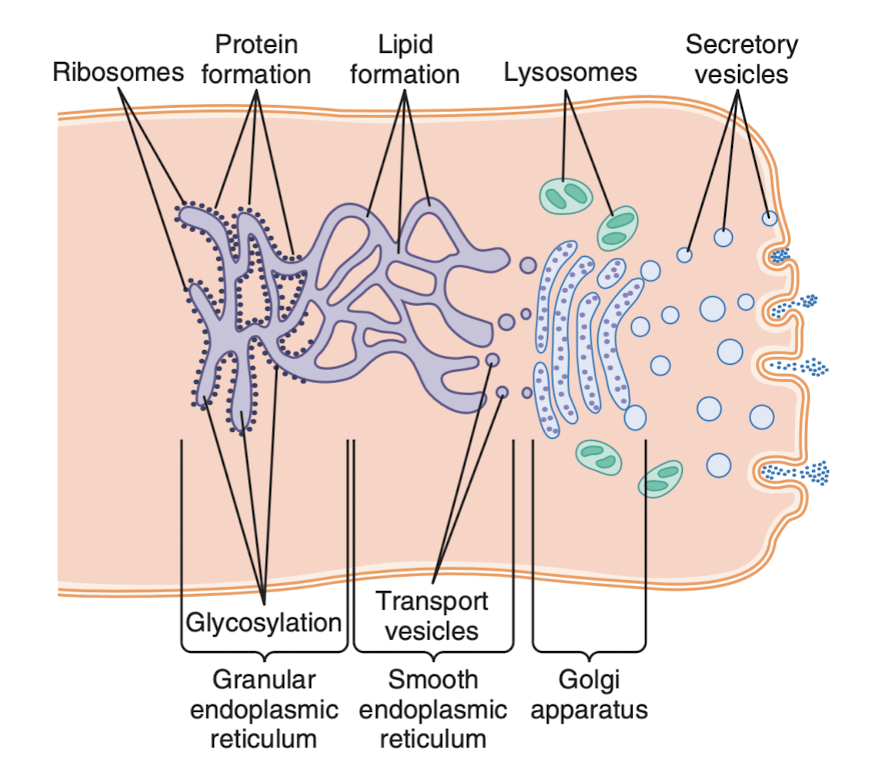
Of course, very importantly: how do we get energy from food? It's all from things we consume that can react chemically with oxygen: carbs, fats, proteins. Basically all carbs are converted into glucose by the digestive track (and liver!) before they go into the bloodstream. Proteins into amino acids, fats into fatty acids. Then, the mitochondrion inside those other cells, "powerhouse of the cell", as high school biology classified it, turn it into the basic unit of energy, ATP. Simple inputs: O2, glucose, and outputs are basically just CO2 and water. This process is guided by enzymes (which are just proteins that are made in such a way that they can be a catalyst for a chemical reaction). The main ways the ATP is used (1) transport things through cell membrane, like push sodium across cell membrane (2) creation of chemical compounds, like creation of protein (3) mechanical work, like muscle contractions.
Skeletal, cardiac, and smooth muscle make up almost 50% of body mass.
Membrane transport is obviously critical, for instance in the renal tubes (a structure inside the kidney), about 80% of its ATP is used precisely for this purpose (makes sense, that's its job).
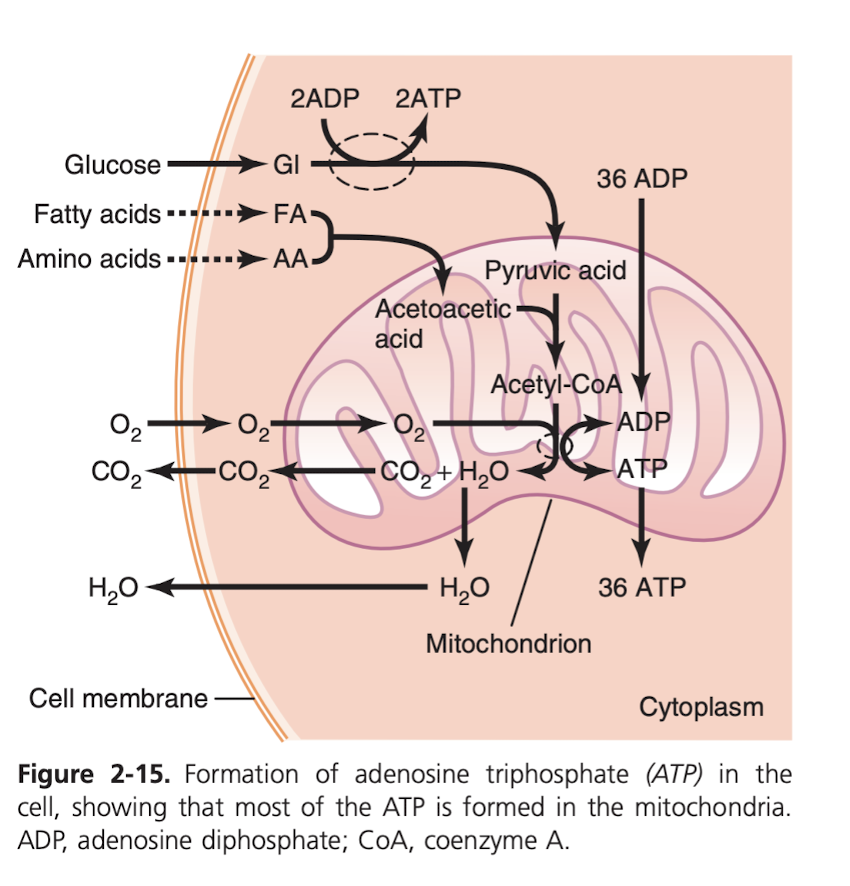
How to cells move around? Ameboid motion (named after amebas, because they move the same way). It's pretty crazy. It kind of makes a conveyer belt for itself that it slides along. The most common cells to use this method are white blood cells, like when they are trying to attack an infection, they need to leave the bloodstream and navigate the tissue to get to the site of the infection. Related to wounds, cells called fibroblasts do some movement, they're what secrete collagen so that that part of the skin can be restored (this is complement, I believe, with skin stem cells, which produce more skin cells to replace the lost ones). How does a cell know to move in a certain direction? We know it's caused by the existence of certain chemical substances in the tissues, but we don't actually know the mechanism for how the cell knows it.
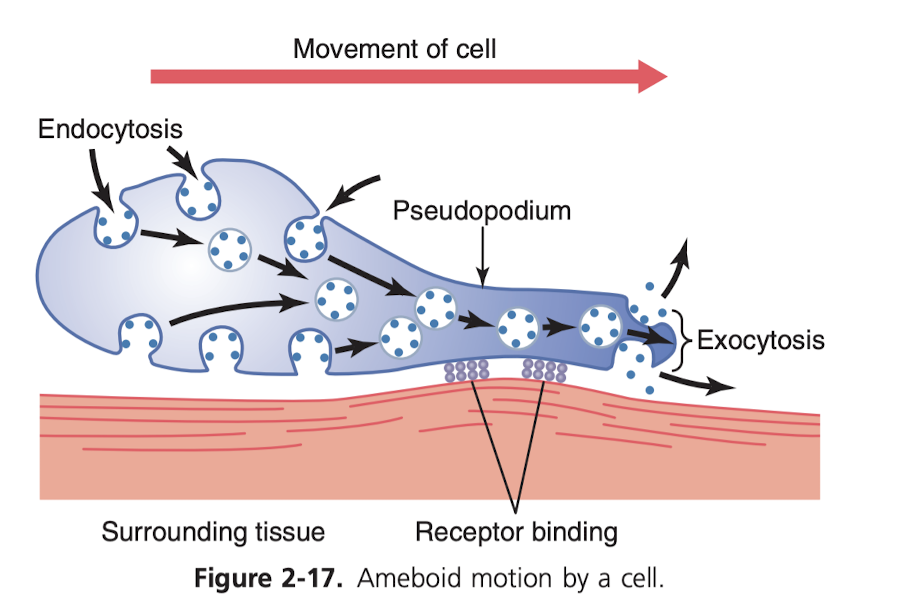
The other type of motion for a cell is ciliary motion (this is similar to how sperm move, it's how respiratory cells move mucus in your nasal passages, it also seems to be how things move in the fallopian tubes as well). The whiplike motion of the cilia on the surface of the cells (as many as 200 on a cell, each about 2-4 micrometers) actually moves the mucus at a rate of 1cm / minute toward the pharynx for destruction.
This is entirely high school biology review, but DNA is transcribed into RNA and then the RNA leaves the nucleus and is used to create the proteins throughout the cell. The proteins are either "structural" or "enzymes", structural meaning that they're like creating new ribosomes or mitochondria or something. Remember A-T, C-G. And each triplet represents an amino acid, and you combine amino acids to make proteins. Easy. Remember ribosomes are really what take the mRNA and string together the amino acids to make the proteins. DNA proofreading is the process taken when the DNA that has split during mitosis is checked to make sure the A in one strand matches only a T, C to G. If there's a mistake, it's corrected. Mitosis is of course cell division.
Some cells last only 10 hours, such as the bone marrow cells, while others like most nerve cells last the entire lifetime of the body.
Remember programmed cell death is called apoptosis. It's becoming clear that abnormalities in apoptosis play a major role in neurodegenitive (like Alzeihmers), cancer, and autoimmune diseases. It seems like chemo works because it induces apoptosis in cancer cells.
Remember that "phag" means "to eat", and so phagocytes and variation are usually responsible for consuming the resources of dead or dying cells. "Onco" refers to cancer.
Cell growth occurs in response to a) growth factors like a signal from a nearby tissue or something, b) until it runs out of physical space c) when their secretions start to pool, which it seems is a feedback mechanism for it to stop. Related to this and very critical are telomeres, which are just repeated base patterns (about 8000 base pairs in red blood cells) at the end of each chromosome. Basically, during mitosis, you can't keep the ends of the DNA, so these act as buffers, and telomerase (the enzyme) adds more buffer to the end after the division to keep the telomere from getting completely deleted. But when you have enough cell division, the buffer can run out, and that the cell becomes "old" or senescent and will stop dividing. That means eventually it'll die, and won't be able to replenish itself via mitosis, so then you just have cell death in organs, in other words, a disease. This is exactly what aging is. Aging and cancer are very intricately linked is the thing, because in cancer cells telomerase is super activated and that allows the telomeres to stay at normal length even after rapid cell division, and so it basically undoes that control mechanism where rapid mitosis would eventually cause cell death because the telomeres would get too short and cell death would ensue.
Cell differentiation is a pretty fascinating thing as well, because obviously every cell has the capacity to be anything. It seems like in the embryo, some certain fancy stuff is going on at first, but then another really interesting thing happens. Basically when you have the eye cells coming into contact with the head, it causes the lens of the eye to form. So it's not like the cells know they just all of a sudden need to stop making the eye and start on the lens, it's that they come in contact with the head and then that causes the change.
Cancer is rare, and that's because of how many control mechanisms exist to prevent it. Lots of stuff has to go wrong. 1. Most mutated cells that would be cancerous just die because they mutated all wrong 2. only a few of the ones that do survive become cancerous survive because most mutated cells would just run out of telomeres and stop growing 3. cells that would be a cancer can produce funky proteins and the immune system recognizes that and kills the cell. 4. it's not usually a single gene mutation, it's multiple genes that have to go wrong (for instance you might have rapid cell growth, but no gene that allows blood vessel growth, so that growth dies because it doesn't get nutrients). Despite all this, probably one in every few million still has significant mutant characteristics.
So what causes cancer? Mutations, of course. But those can occur at increased rates if you're exposed to ionizing radiation (x-rays, gamma rays, radioactive substances, etc.), specifically this radiation causes parts of the tissues to ionize (knocks off or adds an electron), which makes the atom much more likely to kind of fuck around and react chemically with other things (obviously, because of its charge), and it can actually rupture DNA strands causing many mutations. Also of course carcinogens (which are just certain chemicals, most commonly cigarette smoke which causes about 25% of cancers). Physical irritants can also cause it, like constant abrasion of certain foods in the intestinal track (or in my case GERD causes the acid to erode the lining of the esophagus) which results in rapid and constant mitosis to replace these cells, which increases cancer risk. Then there's heredity, because some genomes (from your parents) may already have some mutations that mean you only need one or two for a cell to become cancerous, whereas a "healthy" genome would need four or five mutations, so it's just more likely for you. Finally, viruses actually can cause cancer, because they modify the DNA of your cells directly or via RNA "reverse transcription" and bam, you've got new DNA with cancer. Another thing to note is that cancel cells are less adhesive to neighbors, so they wander around tissues and shit and enter the blood stream which is really bad because then they spread all over the body (metastasize).
Why does cancer kill? Pretty simple, the cancer cells just use up all your body's resources and ultimately result in cell death in everything else.
Really just two ways things get through the cell membrane: diffusion (uses the heat energy, i.e. the normal molecular movement of the molecule through the membrane; will go from high to low concentration) or active transport (uses ATP, actual energy to transport).
Diffusion can happen, depending on the molecule diffusing, even without specific gates that they pass through the membrane. The bilipid layer (two fats basically pointing at each other (below)
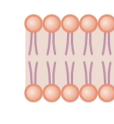
is impermeable to a lot of things, but oxygen for instance can diffuse through it naturally. That's great, because it means you don't have to use any energy to make sure you've got a good balance of O2 in each cell. Remember that the reason things tend to equal balances inside and out when diffusion is occurring is just because of probabilism: things are bouncing around and if you have 2x as much O2 outside a cell as inside a cell then on average you'll have 2x as much crossing inside than going outside, until eventually things even out.
Then you can have diffusion happen facilitated by gates, like in the case of sodium for action potentials in nerves. Cells in general are going to have a negative potential, about -90 millivolts, compared to the outside, and there's a lot more sodium ions (NA+) outside the cell than inside, because of various pumping mechanisms that the cell does, and at certain points, explained later, a gate that's specifically permeable to NA+ will open and due to the higher concentration outside the cell _and_ the negative charge inside the cell, the positive NA+ ions will diffuse in faster than they'll diffuse out.
Then of course you have more active transport which goes against the diffusion gradient, which takes actual energy (ATP) from the cell to do. This is really more on a molecule by molecule basis.
You also have voltage gating, which is basically where the gates only open when certain voltages are hit inside the cell. This is why the gates open in the case of sodium explained above.
There's also facilitated diffusion, where a molecule to diffuse will bind to another molecule to allow it to pass it through a gate. One of these things that in transported facilitated diffusion is glucose. Glucose transporter 4 is one of the things that allows glucose to diffuse, and it's actually activated by insulin, which increases the rate of facilitated diffusion as much as 10-20x, and this is the primary way insulin controls glucose use in the body.
Then you have chemical gating (also called ligand gating), which is where a gate will open only when a certain substance binds to the protein controlling the gate. This is also on a molecule by molecule basis, but one great example of this is how nerve cells cause muscle contraction. Basically, a neurotransmitter is released by a nerve cell that causes a muscle cell to open a gate that allows sodium to pour in, thereby initiating an action potential in the muscle cell. Basically it's like the interface between two different types of cells, and it uses a neurotransmitter to open a specific gate to transmit the "signal" (i.e. action potential).
Sodium/potassium pumps are critical for controlling cell volume. It pumps three Na+ ions out for every two K+ ions in. Apparently this charge somehow causes osmosis of water into the cell, and so it would swell infinitely if this pump didn't do its job (?).
Examples of active transport for hydrogen ions: increased in the stomach to 1 million times concentration and combined with chloride to form hydrochloric acid in the stomach. Also secreted into urine against concentration of 900-fold to pull hydrogen ions out of the blood and into the urine (kidneys).
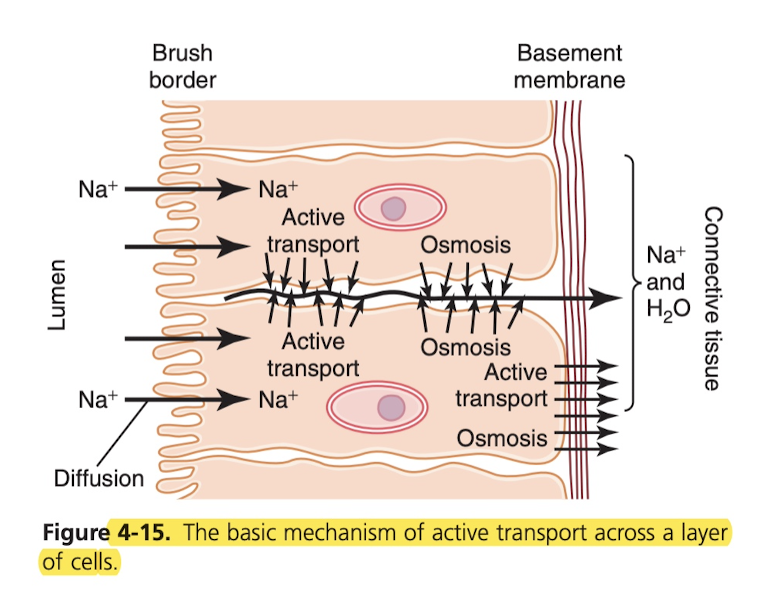
This is how nutrients, ions, etc are absorbed into the blood from the intestine. The brush border is permeable to water and sodium ions, so you get that for free (diffusion). Then inside these long cells, the cells do active transport to push the Na+ ion outside the cell into the connective tissue, and water follows for free (I suppose due to the charge of the Na+).
The method of action potentials is rather simple. Basically something, explained later, causes the voltage to rise in the cell to a certain level (usually small increases in Na+), and once it hits a certain voltage, voltage-based sodium gates open and let in even more sodium ions, they just pour in. These gates actually just stay open, until the voltage gets back to that close to resting level. Basically the sodium gates is a positive feedback loop, where once the charge rises more opens, which causes the charge to rise more which means more opens. Then after a certain point the potassium channels open due to the high charge, and so potassium starts to pour out due to diffusion (remember, extracellular has high sodium, intracellular has high potassium concentration), which normalizes things.
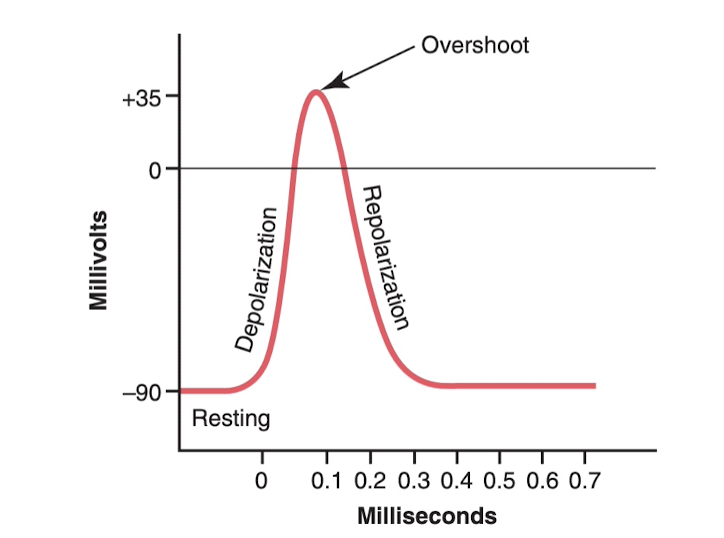
Remember that action potentials travel in all directions, and it basically just starts in a region and traverses the length of the axon/nerve fiber/wherever it's happening. The transmission of this depolarization is called a "nerve/muscle impulse".
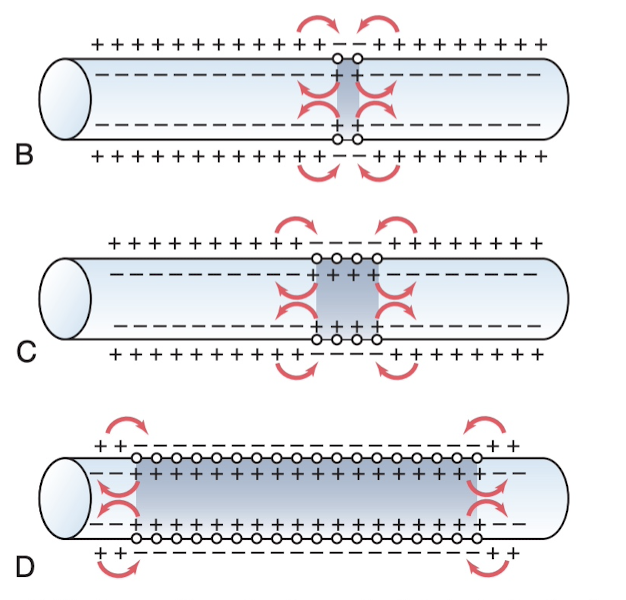
This same process of depolarization/repolarization can happen with calcium ions and calcium gates as well, but it's a much slower process than the sodium action potentials, which may be due to the concentration of calcium ions in the extracellular fluid, but not sure. (?)
Calcium ions are critical for normalizing action potentials (we're not sure why, but it's likely because calcium ions bind to sodium channels, and the positive Ca2+ ions alter the gate such that it changes the voltage required to open it). If Ca2+ ions fall below 50% normal level, the sodium channels become very sensitive to changes in membrane potential changes, which can cause spontaneous discharge of action potentials, which can trigger muscle contractions. This is called "muscle tetany", which can be fatal due to tetanic contraction of the respiratory muscles.
What causes the heart to beat rhythmically? Peristalsis to occur in the intestines, and breathing to occur rhythmically? The answer is: the membranes must be a little extra permeable to sodium. This makes it so the resting membrane potential is only -60 or -70 millivolts, rather than -90 like most of the others. Then what happens is just kind of a bouncing effect: some sodium comes in, feedback loop starts and action potential occurs, potassium brings it back down again (repolarizes) but the levels are close enough that spontaneous action potentials can cause again. This happens at a regular interval. But the main question is, why the 1 second dealy? Well that's the potassium gates opening up and being a bit slow to close, which means you "overshoot" in the other direction, gradually, and then they start to close again. As always, it's a matter of action and counteraction.
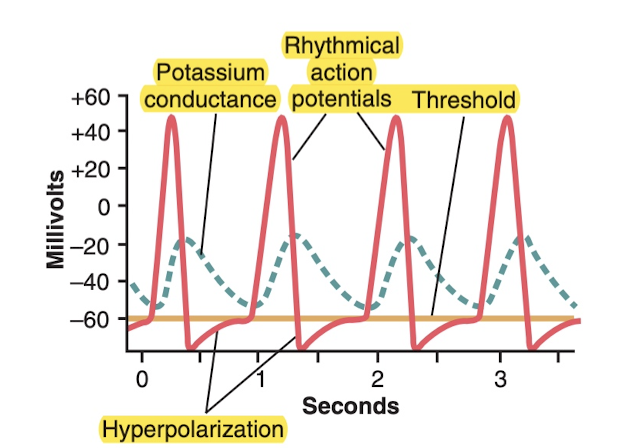
Explained in more depth later, but basically the action potential travels along the nerve cell until it reaches the terminal point, which is embedded in a bunch of muscle cells. When the action potential reaches the terminal, there are calcium ion voltage-gates there, and that causes them to open, Ca+ pours in, which in turn causes a neurotransmitter called acetylcholine to pour into the synapse, which in turn bind to channels on the muscle cell which cause them to open, which allows sodium ions to pour into into the muscle fiber, which in turn depolarizes (i.e. induces an action potential) in the muscle cell with travels along the muscle and (via a mechanism explained later) causes it to contract.
Neuron is an axon, covered in a myelin sheath which has little divots every so often that expose the axon to the extracellular fluid. This divers are called the nodes of Ranvier. This is actually pretty good, because only the nodes are what have to depolarize/repolarize, rather than the entire length of the axon, which apparently would be about 100 times more ions than would be necessary (much less energy through active transport).
But what actually causes the action potential? Depends on the neuron. Can result from mechanical disturbance (pressure to excite sensory nerves in the skin), chemical effects on the membrane (transmit signal between neurons in the brain), or passage of electricity through the membrane (transmit signal between successive muscle cells in the heart/intestine).
Local anesthetics, such as procaine and tetracaine, act directly on the sodium gates, making them much more difficult to open, which reduces the excitability of the neurons to basically zero, so nothing can trigger pain there.
There are three types of muscles: skeletal (basically all the ones you usually think about, like your biceps, quads, etc) which makes up 40% of the body, and then about 10% is smooth muscle (throat, blood vessel walls, etc) and cardiac (heart).
Tendons: are just some fibers that connect the muscles to the bones so that they can act as levers.
How muscles work: You've basically got the muscle, which is made up of man muscle fibers, which in turn is made up of several hundred/thousand myofibrils. Each myofibril is made up of ~1500 myosin filaments and 3000 actin filaments. It's these small things that are responsible for muscle contraction.
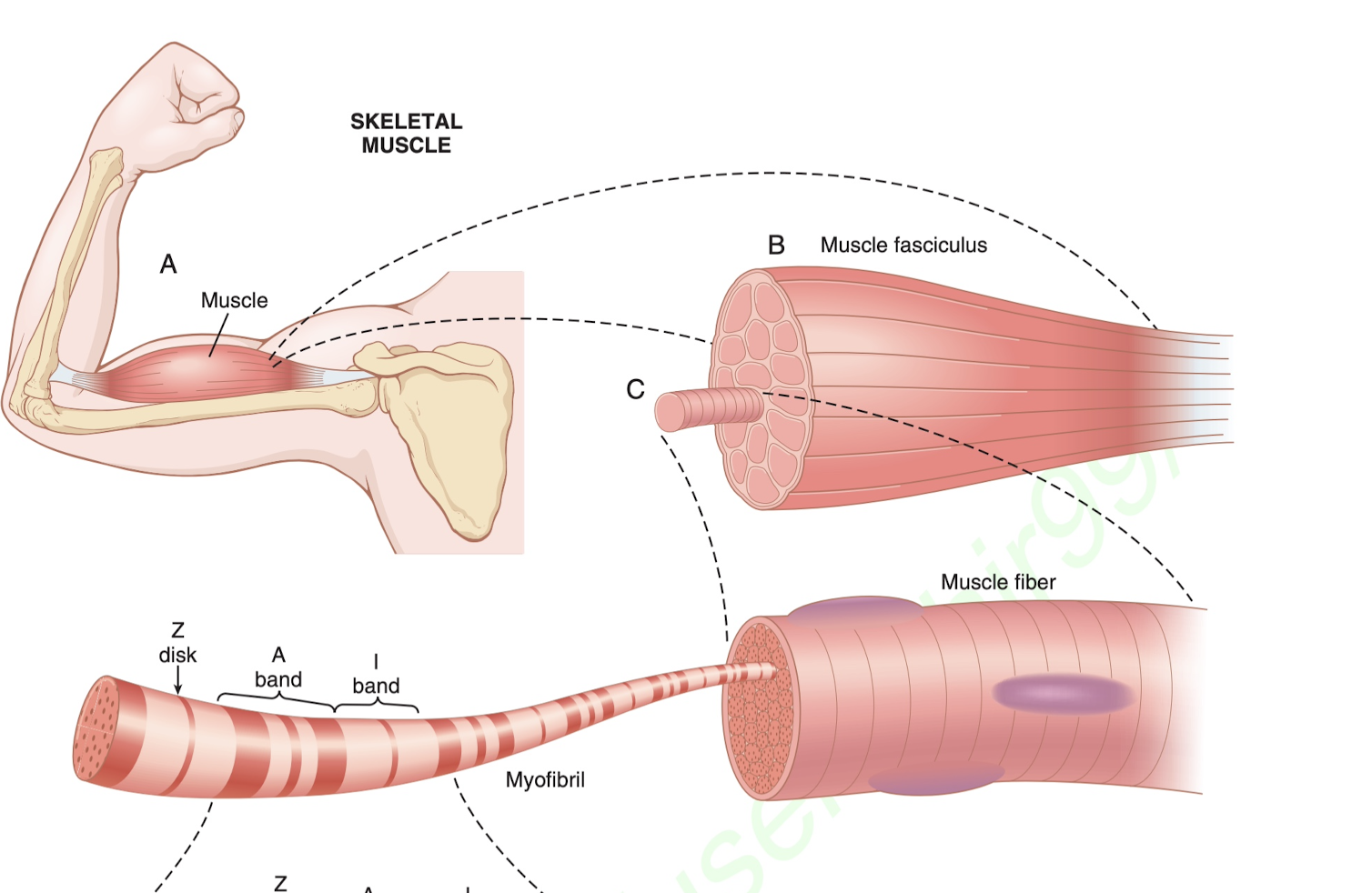
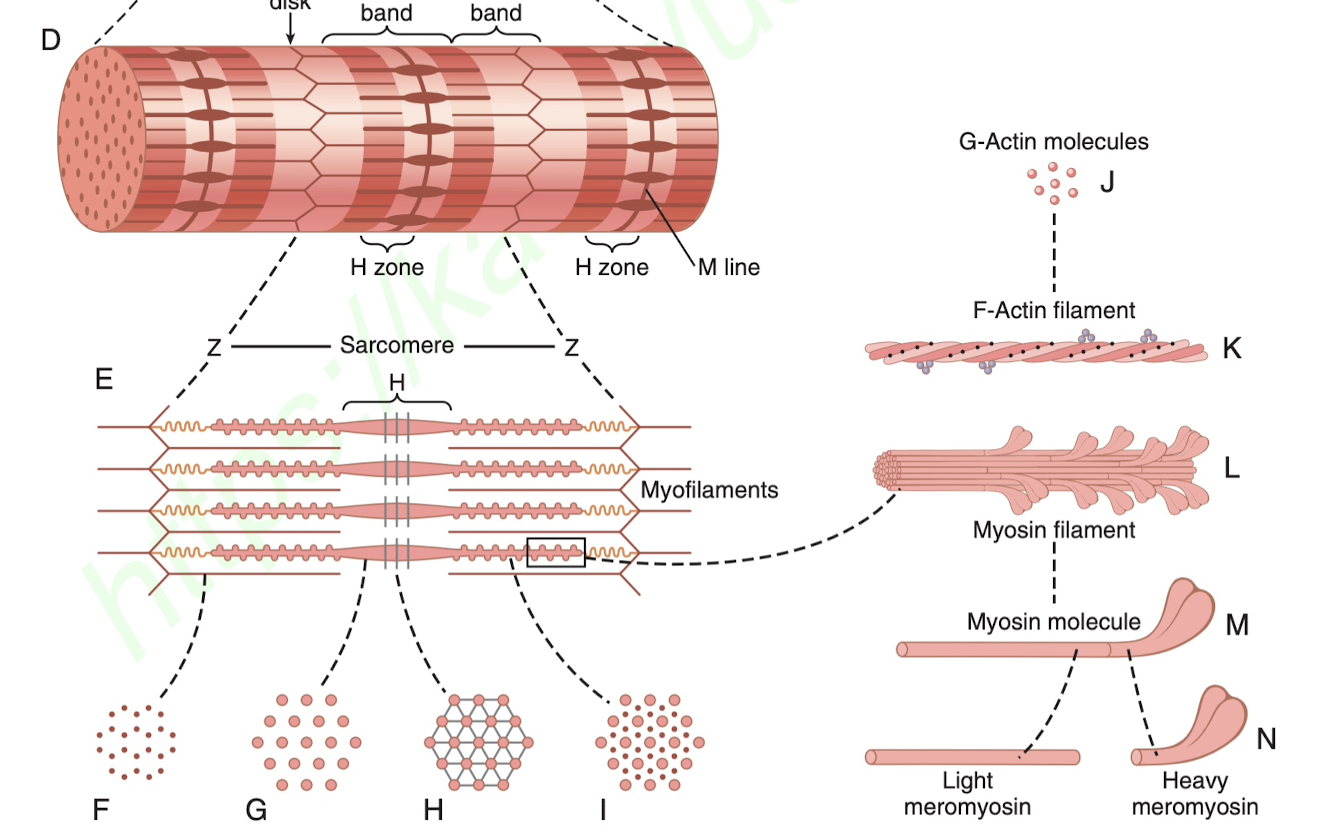
Fundamentally, how the muscles contract and also extend beyond the resting state is on the smallest level those myosin and the actin filaments.
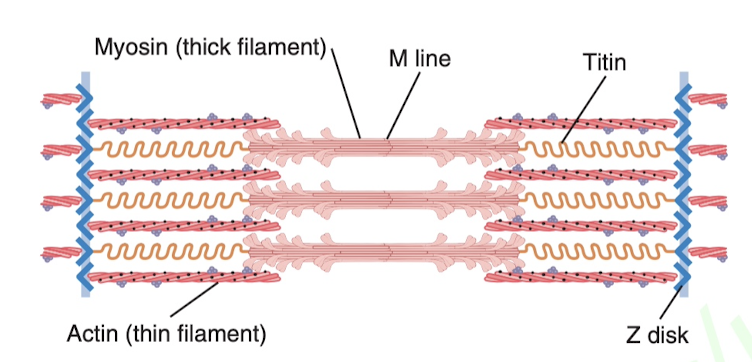
Between two Z disks you'll have a setup like this. Imagine the titin as a spring to keep everything in place, then you have these little knobs on the myosin that actually are like little levers that can pivot (called cross bridges), and basically when they pivot they pull such that the myosin overlap more (contract) with the actin or less (extend). The specific cellular mechanisms are explained more below.
Contraction process: Action potential travels along a motor nerve to its ending on muscle fibers, which causes the release of the neurotransmitter acetylcholine in the synapse between the motor neuron and the muscle. The acetylcholine binds to sodium gates allowing them to open (an example of chemical gating, above) which causes Na+ to pour in, which leads to an active potential in the muscle, which travels along the muscle fiber. This action potential in turn causes the release a lot of calcium ions (this time, via voltage gating) which causes the nubs on the myosin filaments to become attracted to the actin, which basically initiates (in combination with ATP) the crawling of the myosin which is actually like pulling the two Z disks closer together. The more cross bridges in contact, the greater the force of contraction. The Ca++ ions are pumped out immediately (and the acetylcholine is rapidly removed via acetycholinesterase causing the sodium gates to close) and contraction ceases, until the next impulse is received (you have to send repeated impulses to get things to continue). Very interesting: nicotine has nearly the same effect on muscle fiber as does acetylcholine, except it's not actively destroyed/it's destroyed slowly enough that the action potentials persist for minutes or hours. Conversely, diiosoproprly flurophosphate (a powerful nerve gas poison) inactivates acetycholinesterase for weeks causing contractions to never cease, inducing death.
The space between the myofibrils (called sarcoplasm) is filled with a bunch of potassium, magnesium, and a ton of mitochondria.
Where does the energy come from for cells: Now for where this energy is coming from. Basically, 3 types of stored energy. You've got the really fast, ATP, which only enough exists to maintain full contraction for 1 to 2 seconds. Then what's used to reconstitute the ATP (form more of it) is a substance called phosphocreatine, which can pretty easily replenish the ATP. But ATP + phosphocreatine can only sustain maximal contraction for 5 to 8 seconds. The next thing to reconstitute ATP and phosphocreatine is glycolysis of glycogen, which is stored in the muscle cells. This works great, but the unfortunate thing is a byproduct is lactic acid, which is what causes the muscle "burn". One great thing is that this is an anaerobic process, meaning glycolysis doesn't need oxygen as an input. It can last for a decent bit longer, maybe even up to more than a minute. It's also much faster, 2.5 faster, than final step that involves reacting oxygen with food stuffs. The final one: oxidative metabolism (i.e. cellular respiration, krebs cycle, etc), which is combining oxygen with the byproduct of glycolysis (pyruvate also known as pyruvic acid) with carbohydrates, fats, and proteins to reconsitute ATP. Now this is really interesting, basically your body is trying to reconstitute ATP as fast as possible, but the issue is that it can't run the krebs cycle stuff fast enough under high intensity work (e.g. pump in O2 and pump out CO2, and is limited by mitochondria supply as well), so basically anaerobic glycolysis takes over because even though it's only able to produce 2 ATP instead of about 32 ATP (in the case of aerobic respiration) per mol of glucose, it's able to do it fast enough to keep up. The unfortunate thing of course is the the byproduct is pyruvate, which if enough of it is generated and it's not used fast enough for aerobic respiration, is converted to lactic acid which starts to burn your muscles. It's thought that anaerobic glycolysis is an "ancient" form of energy production that we still have around, from before the atmosphere had sufficient O2.
Lots of factors appear to go into this, but on its surface: for very long maximal muscle activity (many many hours), the greatest proportion of energy comes from fats. But for periods of just 2 to 4 hours, as much as 50% comes from carbohydrates. Want to know exactly how the body shifts and knows to shift between these things (?)
Muscles suck. Even in best conditions, only 25% of the energy is being used, the rest is lost as heat. Maximum energy efficiency is when muscles are contracted at moderate velocity (30% of maximum).
Muscle types: 1 and 2. 1 is "slow fibers", which is red (due to oxygenation basically, because it's used primarily for longer term movements (thus aerobic respiration). 2 is "fast fibers", also known as white muscle. These are muscles better designed for rapid and strong contractions (via glycolysis).
Hemoglobin in the red blood cells is what the oxygen binds to. Myoglobin is a similar protein that is contained in type I (red muscle) and it binds with oxygen to store it until it's needed. 4
The maximum strength of muscle contractions is about 50 pounds per square inch, and since quadriceps have up to 16 square inches of muscle, as much as 800 pounds of tension can be applied.
Skeletal muscle doesn't contract without an action potential, so when you see "muscle tone", that actually is resulting entirely from a low rate of impulses from the spinal cord to the muscle. Tone is really a term that refers to the continual contraction of the muscle by slow but constant nerve impulses.
Basically our bodies consist of a bunch of lever systems. You've got two bones (say your upper arm and lower arm), and there's a bicep sitting on the upper arm, but the tendon connects to the lower arm near the top. By contracting the muscle, making it shorter, you're pulling the lower up closer to the upper arm.
Muscle hypertrophy (opposite of atrophy) increase (increasing size of muscles) is almost entirely from increasing the number of actin and myosin filaments. The manner in which forceful contractions lead to hypertrophy is not known. It has been observed that hypertrophying muscles do have myofibrils that split, but its importance in increasing muscle size is not known.
Another type of hypertrophy occurs when muscles are stretched beyond normal length, and essentially they are automatically lengthened where they attach to the tendons, and similarly they are automatically shortened when the muscle continues to remain shortened for extended periods. Another use it or lose it situation.
Rigor mortis, or the state of contraction that exists after death (for the first 15-25 hours), is due to the depletion of ATP right after death. With no ATP in the muscles, you basically have the myosin/actin bridges stuck together (it takes ATP to separate them / grind them along each other). It’s almost like you’ve got two saws put together and you need energy to lift them to separate them, but you don’t have it, so the muscle is just stuck in whatever position it’s in. After like 15-25 hours (the hotter the room, the faster it happens), the muscles actually basically eat themselves (autolysis) and relaxation happens.
Although skeletal muscle fibers are stimulated only by the nervous systems, smooth muscle (such as in the heart, lining every blood vessel, etc) can be stimulated by nervous signals, hormones, stretch of the muscle, or other ways.
Just like skeletal muscle, for smooth muscle you've got nerve fibers that line the smooth muscle, and they are mostly using acetylcholine and norepinephrine. Now what's interesting is that in some organs, acetylcholine excites and norepinephrine inhibits, and in others the converse is true.
Skeletal muscle action potentials have rapid repolarization (signal/contraction ends), but some smooth muscle actually sometimes has plateaued action potentials, for instance the ureters and under some condition the uterus.
Some smooth muscle, such as that found in the gut, is self-excitatory (doesn't need external stimuli from a nerve). We don't actually know why this happens. Basically you have a sinusoidal wave and then if it hits a certain threshold you get a spike on the wave, that's a slow wave, which they also call "pacemaker waves".
Smooth muscle can also undergo spontaneous action potentials due to physical stretching. For instance when the gut wall is overfilled with stuff (in the intestine), "local autonomic contractions" create peristaltic waves that move the contents away (toward the anus).
A couple of things can trigger vasodilation of smooth muscle: lack of oxygen, excess carbon dioxide, increased hydrogen ion concentration, but also things like lactic acid and increased body temperature. Curious if the increased body temperature could be what helps with headaches when you put something warm on your head, not sure if that's going to be smooth muscle though.
The heart isn't one pump, it's two separate pumps. "Right heart" that pumps blood through lungs, "left heart" that pumps blood through the rest of the circulatory system, providing bloowd to other organs and tissue. Each of these hearts has two chambers, one chamber called an atrium and one called a ventricle. The atrium is just like the "primer", it's pretty weak, and just pushes to the ventricle, which does the heavy lifting. The Atria triggers first, followed soon after the ventricle. That's the bumBUM of the heart beat.
You've got special muscle for each chamber, but then also another type that controls the rhythmic beating of the heart, it's excitatory muscle. In some ways, the muscle of the heart is very similar to skeletal muscle (as opposed to smooth muscle), but in other ways, it's vastly different. For instance, cardiac muscle is what's called a synctium, which is basically a bunch of cells fused together such that one being excited rapidly spreads to the others, so that they almost act together in unison.
There is basically a limit to one's heart rate. Based on the refectory period of the muscle contracting, and that's about 0.25 seconds, so 60/0.25 seconds is 240 beats per minute.
A heart placed in a calcium-free solution will stop beating. You need calcium for the heart to beat, it's critical to the triggering of the contractions.
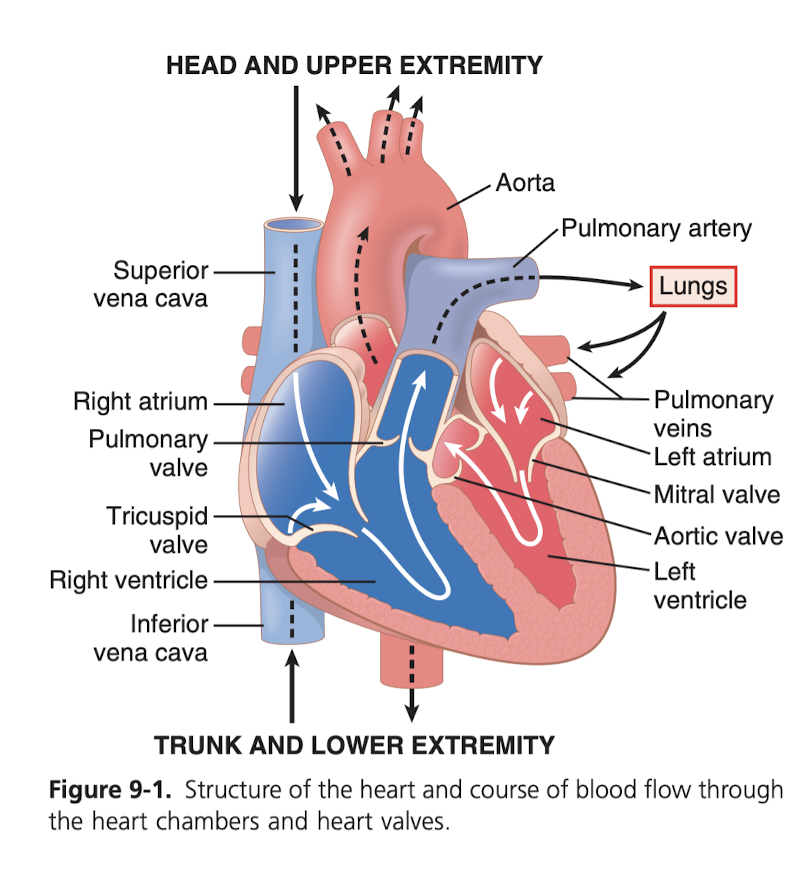
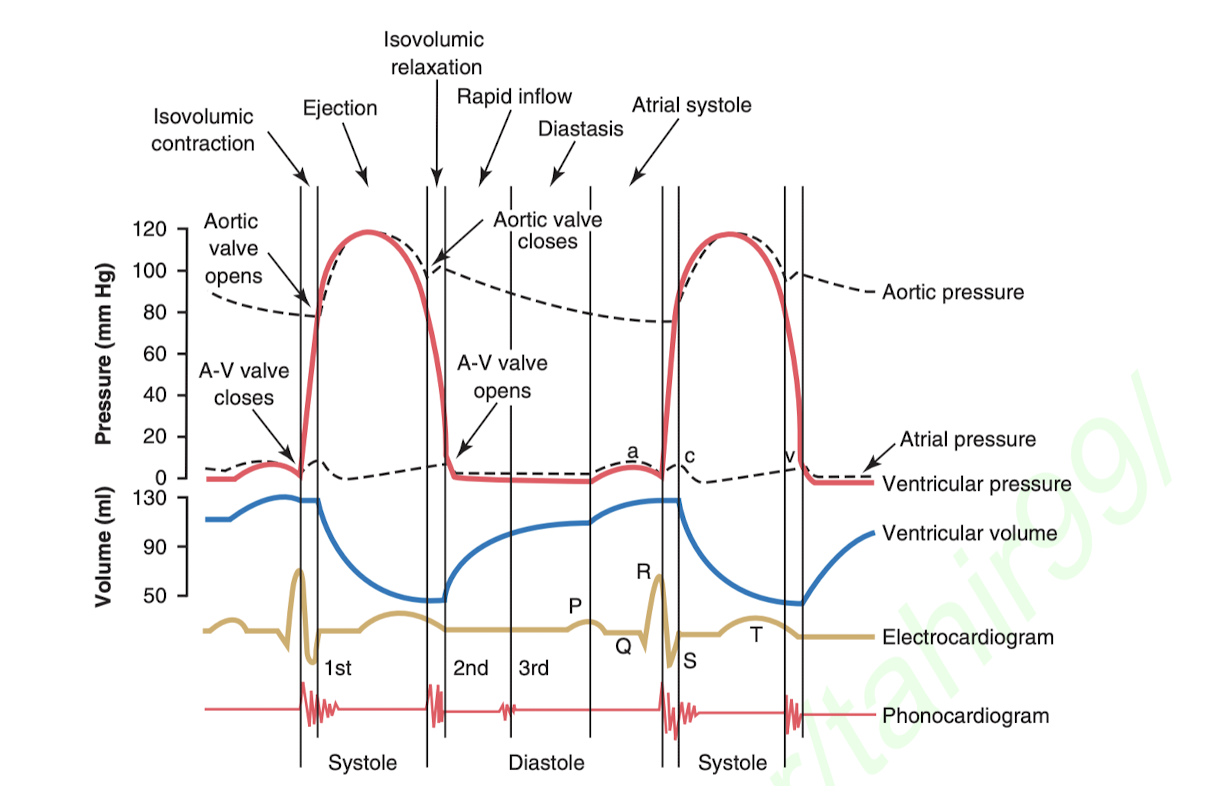
Systole is contraction, and diastole is relaxation. During systole, the ventricles contract, during diastole the ventricles then relax during diastole, during which they fill with blood from the atriums. Diastole is a long while because the blood needs to fill the big ventricles, and systole is shorter because it’s the period of rapid contraction of the ventricles which shoots all the blood out. Should think "systole", "short", "contraction". "Diastole", "long", "filling". Lining this up with the phonocardiogram (the sounds), can be counter-intuitive. It's "lub", "dub", with the dub being louder and higher pitch, this is because it's the bigger valves closing, and it's the bigger valves closing because the ventricles just finished supplying blood to the body/lungs. "lub" starts systole (short timeframe, small sound because small valves are closing, small depolarization, atria contract, then and large ones are opening, large depolarization, ventricles contract, big ejection of blood) and "dub" starts diastole (long timeframe, large sound because large valves are closing and small ones are opening, everything relaxes and blood starts pouring in from the vena cava).
About 80% of the blood that’s pumped by the ventricles fills during diastole with blood pouring in directly through into the atria and through the valve to the ventricles, and then the last 20% comes from the atrial contraction to force a bit more in before the ventricular contraction.
A heart rate isn't great, because the the chambers can only fill so fast. So a very fast heart rate means that the chambers aren't completely filling before the next contraction.
The diagram above is critical. It should be really closely studied.
You'll see that the electrical signals, measured by the electrocardiogram, happen slightly before the actual contraction/relaxation, because they're causing them to happen.
Remember artery means going away from heart, and vein means going to the heart. But just because it's an artery doesn't mean it's oxygenated, for instance pulmonary artery has deoxygenated blood going toward the lungs and away from the heart.
The Sinoatrial node is the "pacemaker", and it's what's responsible for initializing the contraction. Signal travels to the Atrioventricular node (AV node), which introduces the delay between the atriums contracting and the ventricles contracting, so that the ventricles can be primed.
The Aorta is a really big tube at the top that sends the oxygenated blood to the rest of the body. The two coronary arteries which are arteries that splits off of that big tube and provides some of that blood to the heart itself so that it is getting a supply so it can actually power itself.
If you hear more than a lub and dub, that’s probably a problem. It means the valves aren’t closing in unison.
When the heart muscle is dilated due to disease, it requires more time+chemical energy basically to contract, which is bad because you get into a positive feedback loop where it becomes less able to deliver energy which makes it more dilated etc.
4-6 liters of blood is pumped each minute. I think actually there is only about 4-5 liters of blood in a human, so you do a full cycle every minute at rest. During, it’s 4-7x higher. The amount of blood pumped for a given person is determined almost entirely by how much is actually returned from the veins, called venous return.
Potassium is discussed and how levels too high can cause aryhrmias in the heart, and even stop it. As suspected this is what is used to stop the heart in lethal injections, simply potassium. Basically you’re eliminating the membrane potential for the heart muscle, which prevents an action potential from being triggered and therefore contraction to stop.
Increased body temperature apparently increases heart rate by a significant degree. And decreased means lower heart rate. It’s believe that this is because increased temperature means increased ion permeability and so it’s easier to trigger the action potential (lower threshold I assume), which means the cycle is smaller.
So much of the body is just careful creation of certain muscle that has varying permeability to certain ions and has other properties. For instance, the Sinus node in the heart which is basically he pacemaker of the heart, is more permeable to sodium so it has a higher resting potential than surrounding muscle, this means certain sodium gates are inactivated, which causes it to take more time to "charge" before an action potential and "discharge" after one, and it's in a constant cycle of doing so, just on its own because its leaky enough to sodium that as soon as the action potential is over the sodium starts slowly coming back in, rising until it hits the action potential threshold. It is just going in a constant circle, and it initiates the beating of the atria, which travels to the ventricles via the AV node, and causes the ventricles to contract, and then the cycle continues. You've also got a fibrous barrier which acts as an insulator, between the atria and ventricles (preventing atrial contraction from causing ventricle contraction; it must be done via the A/V node, which introduces the delay). Could have serious arrhythmia's if the muscle does penetrate this insulator.
I’m curious: could I have an abnormally low resting heart rate because I have a messed up sinus node? Could it have just reverted to some of the other methods for spontaneous action potential generation?
Interestingly, there are other parts of the heart that are also self-exciting, like the AV node and the Purkinje fibers (which are the fibers that spread the signal out all over the ventricles). The thing is, that they both are lower rates of self excitation, so the sinus node "wins" because it's cycling everyone the fastest. But in abnormal hearts, there's an "ectopic" pacemaker, which is when some other part of the heart is dominant (self-exciting faster than the sinus node). Obviously the BPM might be similar or the same, at a resting state, but the issue is the _direction_ that the "beating" travels; it might originate in the ventricles instead of the atria, or some other crazy shit. This means a much worse ability to actually pump blood.
Very interestingly, you can have an AV block, which is when the signal doesn't pass between atria and ventricles via the AV node. If this occurs suddenly, the "backup generator" of the Purkinje fibers, which beat between 15 to 40 bpm, doesn't kick in for 5 to 20 seconds later. Here's the crazy part: during these 5 to 20 seconds, the ventricles obviously aren't pumping. It only takes 4 to 5 seconds for the person to faint because lack of blood flow to the brain. This delay is called Stokes-Adams syndrome.
Parasympathetic (also called Vigal) seems to mean "slow things down" and sympathetic seems to mean "speed things up".
The heart actually sits in a sack, called the pericardium, or pericardial sac, which prevents it from being interfered with by other organs, protects it from infection from other organs, lubricates it, etc. When you get shot in the heart, you usually die from one of two things: massive hemorrhaging (losing blood) or cardiac tamponade, which is where this sack fills up with blood from the heart and restricts it from pumping.
ECG is rather simple. When depolarization of the cardiac muscle is occurring, that current is actually detectable on the surface of the skin. The "width" of the wave indicates how fast the depolarization/re-polarization is occurring. The magnitude of course is the "size" of how much voltage there is, basically. By looking at these waves, you can infer what is going on with the heart. Maybe the magnitude is too small, too high, or parts of the waves are elongated or shortened. Each of these leads to different conclusions. It’s important to note that part of why this is possible is because there is a directionality to the depolarization. The sinus node is on the right heart, so when you put an electrode on that side of the heart, it becomes more positive (?) compared to the electrode on the other side and that potential difference is exactly the wave you’re seeing in the ECG. This is why the Apple Watch requires both hands, why the ECG machines require the electrodes on opposite sides of the body, etc.
"Septum" just means a partition separating two spaces. Two examples is the part of the heart separating the ventricles, and also the partition between your nostrils.
Skimmed through part of chapter 12, it's just too in depth on the vector analysis for the ECG.
Very interesting, so if you have high blood pressure your left ventricle (that pumps oxygenated blood into the body) hypertrophies (gets stronger/bigger), because it has more resistance to the flow and so it needs to be stronger. This increased muscle mass also means a larger magnitude on the ECG.
Remember "left"/"right" for the heart is relative to the person, so if you're facing the person, their left ventricle is on your right.
Myopathies means "disease of the muscle". So Cardiac Myopathies is essentially heart disease.
Hypoxia is: deficiency of oxygen reaching tissues. Hypo is "too low", hyper is "too high". e.g. Hypothermia.
Having magnitudes on ECG that is too low can indicate a variety of things. It could be that there's decreased muscle mass, because of prior "myocardial infarctions", which means "death of heart muscle due to circulatory blockages", basically, translated, which is colloquially known as a "heart attack", which by the way is different than "cardiac arrest", which is much more serious, which is when the heart stops entirely (an electrical problem), as opposed to a myocardial infarction (circulation problem). Anyway, it could be because of prior heart attacks that caused muscle loss, it could extra fluid in the pericardium, as discussed earlier, this is actually because it causes the signals to "short circuit" because they conduct very easily through this fluid, resulting in less of a voltage between the two ECG leads (clips thingys).
"Effusion" means the escape of fluid into a body cavity, by the way, so you can describe maybe getting shot in the heart causing "pericardial effusion", because the blood accrues in that cavity. Pleural effusion is the build up of fluid in the lungs (it also can cause the short circuit just as pericardial effusion can). Another interesting cause of reduced voltage on the ECG is pulmonary emphysema; one of the consequences is more air in the lungs, which acts as an insulator compared to healthy lungs, again reducing the voltage.
During acute coronary thrombosis (which, could progress to myocardial infarction "heart attack"), apparently often the heart muscle does not die from ischemia because the blood flow is sufficient enough to sustain life but not enough to repolarize (and therefore contract). This is good, because it means you don't have myocardial infarction, which I believe is irreparable, so you could make a full recovery after coronary bypass surgery. The "current of injury" is just using an ECG to determine where the blood flow is cut off from. The ECG determines this because that area isn't polarizing, so the location of the injury can be deduced based on the current in the ECG. Usually immediately after a heart attack, an ECG will be done to determine the issue.
Angina pectoris refers to pain from the heart felt in the pectoral areas of the chest, it also radiates to the left neck, and down the left arm. It's usually caused by only moderate ischemia. Usually the pain is not felt at rest, only during overworking of the heart. This is a key point for recognition of a heart attack.
Coronary artery bypass surgery (CABG, pronounce cabbage), also known as bypass surgery is to restore heart function when a coronary artery is blocked. Two approaches: take the internal mammary artery, basically an artery that supplies blood to the left breast) and redirect it to right after the block in the coronary artery to supply blood (preferred) or take an insanely long vein from the leg (great saphenous vein, which goes from the big toe up to the inner thigh) and detach it in both ends, and attach one side to the Aorta and the other to the coronary artery. This is usually performed with the heart stopped (again, using potassium) and with all of the blood going through a "heart-lung machine" instead. This is a technique known as cardiopulmonary bypass, where the machine, known as "the pump", circulates all the blood in the body and oxygenates it. During this process, the blood is also chilled to induce hypothermia in the patient (82-89 degrees). This is discussed on page 269.
The QRS complex, that sequence on the ECG, is pretty important, probably what gets the most attention.
Thrombosis is the clotting of blood in a part of the circulatory system (that obstructs blood flow).
Hemorrhage: an escape of blood through a vessel (either out of the body or out of the circulation into the body cavity).
Proximal: just means "close to whatever you probably think". So like proximal Aorta would be the part of the Aorta close to the heart.
Ischemia (is-skeemia) is the is the inadequate supply of blood to part of the body (apparently especially the heart muscles).
Vagus nerve is a big nerve that comes out of the brain that's responsible for parasympathetic and sympathetic control of breathing, heart rate, and much more.
Ectopic just means an "abnormal place". So like ventricular ectopic foci just means a place on the heart, in the ventricles, that's acting as a pacemaker when it shouldn't be (should just be the sinus node, up in the atrium).
Infarct (in-farked) means basically just dead tissue due to lack of blood supply. So Ischemia causes infarctions.
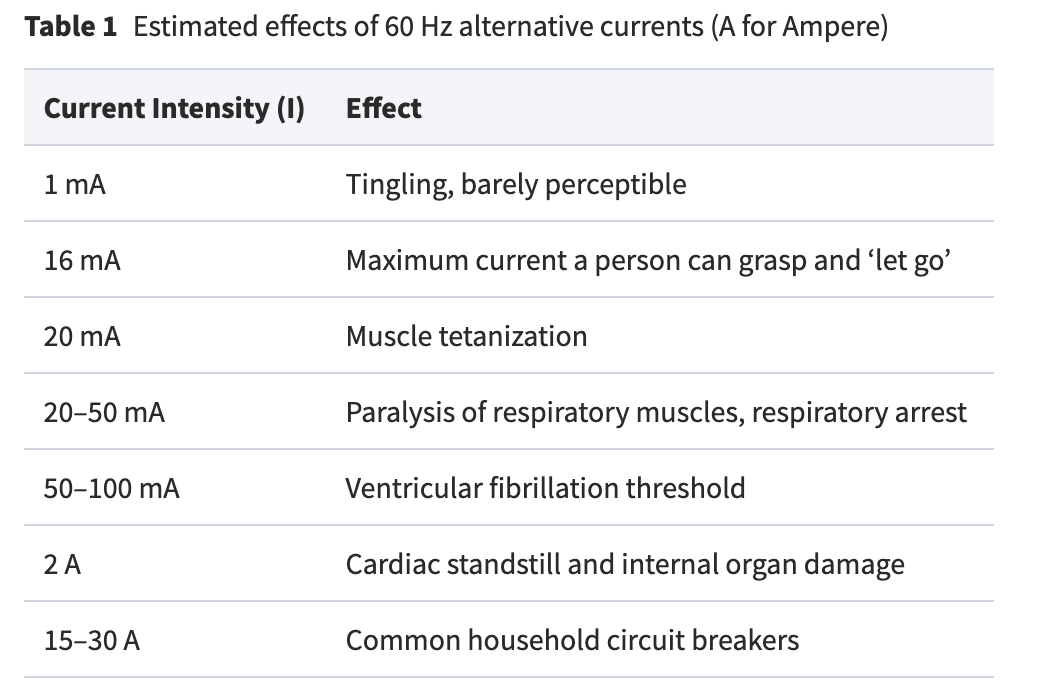
Tachycardia ("tacky-cardia") is fast heart rate (>100/min), bradycardia means slow heart rate (<60/min). Bradycardia occurs in athletes is because their heart is often larger and stronger, so it can pump more blood per stroke. This initiates a feedback mechanism via the arteries that signal to slow down the heart rate.
Heart rate usually increases 10 beats/min for each degree increase in body temperature. So fever causes tachycardia. The reason is simple: increased temperature increases the rate of metabolism of the sinus node, which in terms accelerates the rhythm of the heart.
Stokes-Adams syndrome is very interesting. Essentially, some people have borderline AV node block, it comes and goes. That means they could be fine for a while, and then all of a sudden the block occurs. When that happens it takes 5 to 30 seconds before the Purkinje region of the ventricles to self-exite, so blood isn't being pumped. The person faints in 4 to 7 seconds because of no blood flow to the brain. Then they come back after the ventricles start to beat again. Usually these people are given pacemakers. Untreated, it's a 50% mortality within a year of the first episode.
Very interestingly, premature atrial and premature ventricular contractions (PVC) can both be caused by ingestion of too much coffee (other things can be smoking, lack of sleep, alcoholism, drugs, even emotional irritability for PVC). A high number of PVCs can be really bad. If one happens to happen at a certain period of the wave (end of the T wave), then that could result in spontaneous lethal ventricular fibrillation.
Superior/inferior: just means above and below.
Sinus: just means cavity, in bone or tissue.
Edema: collection of fluid in cavities or tissues of the body. More commonly known as swelling, in some cases I believe.
Lesion: damage on an organ or tissue
Syncope (sin-co-pee): loss of consciousness due to drop in blood pressure. A large enough blood pressure drop means not enough blood to the brain, causing fainting.
Vagus nerve: a rather important nerve, that connects the brain to the heart, lungs, and digestive track. It's parasympathetic control (i.e. slows things down). This is the nerve that's being stimulated (by the brain in most cases, unless maybe pressure is being applied) when vasovagal syncope occurs (below). Its stimulation causes the release of acetylcholine in the heart, which increases permeability to potassium, which hyper polarizes the heart and makes it harder to excite. Result: it decreases the rhythm of the sinus node and it decrease the excitability of the AV junction. Seems like the vagus nerve can also result in vasodilation. You can stimulate the vagus nerve using a "vagal maneuver", to slow your heart rate. Simple easy are holding the breath, coughing, and cold water applied to the face. Deep breathing also does this. Deep breathing triggers blood pressure neurons (baroreceptors) to say "pressure is too high", and so the vagus responds by lowering heart rate. Some people have an excessive form of this, called carotid sinus syndrome. The baroreceptors (pressure receptors) in the carotid sinus region of the carotid artery are extra sensitive, thus even mild neck pressure results in a strong vagal stimulation (sometimes the reflex is so powerful it stops the heart 5-10 seconds).
Vasovagal Syncope: absolutely fascinating. Incredibly common. The vagus nerve above is what's being stimulated by the brain to cause it. It's essentially the parasympathetic overcompensation for a strong sympathetic response (i.e. strain, stress, long periods of standing, heat, the sight of blood). Basically when most people faint, it's probably this. Symptoms are the expected: paleness, nausea, sweating, rapid heartbeat, fainting. It also can cause loss of bladder control during extreme fear (again, overstimulation of parasympathetic). This is what makes a lot of sense: the sight of blood cause is likely evolutionary. If someone cuts you and you see the blood and faint, they'll probably leave you alone because you look dead. Moreover, if you your blood vessels dilate after seeing your own blood (which causes you to faint), this helps slow the process of bleeding out.
Ventricular fibrillation: the most serious of all arrhythmias. If it's not stopped within 1 to 3 minutes, it's fatal. It's pretty simple: when the electric impulses in the heart have completely lost all pattern, and are just circling around the heart, triggering in one place and then traveling around until it's found another place that's underwent re-polarization and triggering there, ad infinitum. This caqusset the ventricles to simply not pump anymore or pumping negligible amounts of blood, meaning no organs are getting oxygenated blood. Within 4 to 5 seconds of ventricular fibrillation, unconsciousness occurs because of blood cutoff to the brain. Especially likely to initiate fibrillation: sudden electrical shock of the heart (at a certain point in the heart rate cycle), and ischemia in various portions of the heart muscle.
Circus movements of ventricular fibrillation: basically meaning 'circle' movements. Essentially if you have an enlarged (dilated) heart, it could make the contraction of the heart muscle be at a slower pace, and if the the pace is slower than the refectory period of repolarization than basically the last part of the heart to contract could then stimulate the first part that already contracted but has now repolarized. This would cause a never ending cycle. Same thing but if the velocity of the conduction is decreased enough (caused by blockage of Purkinje system, ischemia of heart, high potassium, etc). And same thing but if the refractory period of the muscle itself is shortened (various drugs, electric stimulation).
Defibrillation: accomplished via an AED (automated external defibrillator). Although electrical stimulation via AC current can cause fibrillation, a single shock of high voltage electricity for a fraction of a second can save you from this fibrillation. It's simple: it just throws all of the muscles into refectory state at the same time, and then after that's done, a second later the heart should essentially "restart" from scratch and beat on its own. You just place the two pads of the AED on either side of the heart. Unless defibrillation occurs within 1 minute after fibrillation, the heart is usually too weak to be revived from defibrillation alone because of the lack of nutrition from coronary blood flow. This is where cardiopulmonary resuscitation comes in (CPR). Now, this can be done by hand (a doctor opening the chest cavity, and hand squeezing the heart), or without opening the chest by putting pressure on the chest wall. Fibrillating hearts have been pumped for as long as 90 minutes followed by successful defibrillation, so never give up. Lack of blood flow to the brain without this method for more than 5 to 8 minutes usually causes permanent mental impairment or even destruction of brain tissue.
Atrial fibrillation: same thing as ventricular, except it's only the atriums. Often caused by dilation of the atriums. Example of a cause is damage on the heart valve that prevent the atria from fully emptying into the ventricle. Just like with the ventricles, this dilation can lead to slower contraction which if slow enough can result in fibrillation. But even when this is the case, because the atriums are just kind of "topping off" the ventricles, you only have a decrease in 20 to 30% blood pumping, which you can live with for years. When the atria are fibrillating, imputes arrive at the ventricles through the AV node rapidly and irregularly, but because the AV node will only transmit at most every 0.35 seconds, you get a large bound of time when the ventricles might contract, between every 0.35 seconds and every 0.95 seconds, which is a very irregular heartbeat (and the person would probably notice this). The ventricles during atrial fibrillation usually are between 125 and 150 bpm.
The plasma that filters through the blood capillaries into the interstitial fluid does not contain red blood cells or platelets as they are too large to pass through.
Reticular: network-like structure/pattern.
The pulse on the neck is you touching the Carotid (kar-ot-tid) artery, the main artery supplying blood to the brain via the neck. Looks like you have two, one on each side of the neck, and it branches into an internal and external one (each of them has an external and intern one).
Systemic/peripheral circulation is what supplies blood to all tissues (except lungs, which is pulmonary circulation). Circulation is pretty basic, you've got arteries that have blood under high pressure (leaving the heart) going toward arterioles which are smaller branches then leading to capillaries (very thin wall with holes) which is where the fluid/nutrients/etc are diffused into the interstitial fluid. Venules collect blood from capillaries and coalesce it into veins.
84% of blood is in systemic circulation, of that 84, 64% is in veins! Even slight increase in venous pressure causes veins to store 0.5 to 1 extra liter (very flexible walls). When there's severe hemorrhage, it's the veins that will contract a lot in order to keep the amount of blood supply the same as normal to the rest of the body (you can lose as much as 20% of your blood and the body can still function pretty normally due to the reservoir in the veins). Blood traveling in Aorta is 1000 faster than capillaries. Blood remains in capillaries for only 1-3 seconds and gets all its diffusion done!
The reason you die from hemorrhage is because the blood pressure of the blood returning to the heart after going through the circulation is basically 0, and if you lose enough blood, your body can adjust the distribution of blood by constricting veins and such, but eventually there just won't be enough to push a full amount into the heart, which will start to starve the coronary artery, which means the heart starts to fail, which means less blood for the coronary artery, etc. in a bad cycle until exsanguination.
The contraction of your leg muscles as you walk helps reduce the venous pressure at your feet from 90 mm Hg (at rest) to 20 mm Hg (when walking). When standing perfectly still, blood volume leaks under this pressure in the legs and they begin to swell. Within 15 to 30 minutes of standing completely still, between 10 and 20 percent of the blood volume can be lost. This causes fainting in some people and can be avoided simply by flexing the leg muscles/bending the knees periodically.
Varicose veins: large bulbous protrusions of the veins beneath the skin of the entire leg, particularly the lower leg. pretty simple cause. After excessive venous pressure, the veins increase in size and these little flaps/valves that prevent the blood from back flowing (they are directed so it can only flow to the heart) don't increase in size, so they're less effective, which means more blood starts pooling in the veins, which further decreases their effectiveness, until they just don't work at all. When people with varicose veins stand for more than a few minutes, the pressure in the legs becomes very high because of the amount of blood pooling there, and fluid leaks into the interstitial spaces (edema). It can be helpful to have compression socks or elevate your feet when this happens.
During high activity, some tissues need 20-30 times as much nutrients as when at rest. But cardiac output can only go four to seven times resting levels. How is the gap made up? The circulation will dilate/constrict the relevant vessels to ensure a more rapid supply of blood flow.
Ohm's law dictates the rate of blood flow (F = delta P / R) where F is blood flow, delta P is the difference in pressure, and R is the resistance to flow (due to friction between the flowing blood and the cells lining the blood vessel, called the endothelium (the cells that COVID affected)). Imagine the blood traveling through the vessel, and think about how the blood near the center of the vessel isn't being impeded by the friction of the vessel wall, and everything in between is traveling in a speed gradient.
Blood pressure: measured in (millimeters of mercury, that's the mm Hg; when one says pressure is 50 mm Hg, it means that pressure is enough to push a column of mercury against gravity 50 millimeters high). Top pressure (systolic pressure) is usually 120 mm Hg. Lowest point of pulse is usually 80 mm Hg. Two main factors affecting it: stroke volume of heart, how flexible (distensible) the arteries between the heart and the point of measurement is. The bigger the difference between the numbers, that usually means the bigger the stroke (more blood per stroke). The absolute value of the low and the high is mainly based on how distensible the arteries are (how hardened they've become), arteriosclerosis is the term for them hardening, it's caused just by aging and build-up presumably. Blood pressure can sometimes rise to twice normal due to this. How it's taken is awesome. Basically you take the cuff and it compresses until there's no more blood flow through the brachial artery. Then you release the pressure, and as soon as you hear a sound it means that systole was sufficient to shoot the blood through the mostly closed vessel, which means the pressure of the cuff about equals the pressure of the blood. During diastole, the vessel closes again because the pressure isn't as high, so you hear the sound again each time blood jettisons through. Then eventually when you no longer hear any sounds, you've hit the pressure of diastole because the vessel isn't being obstructed in any way and so no blood turbulence is occurring and making sounds. That's how they're measured. It's not a great measurement, but it's within 10% of a direct catheter measurement.
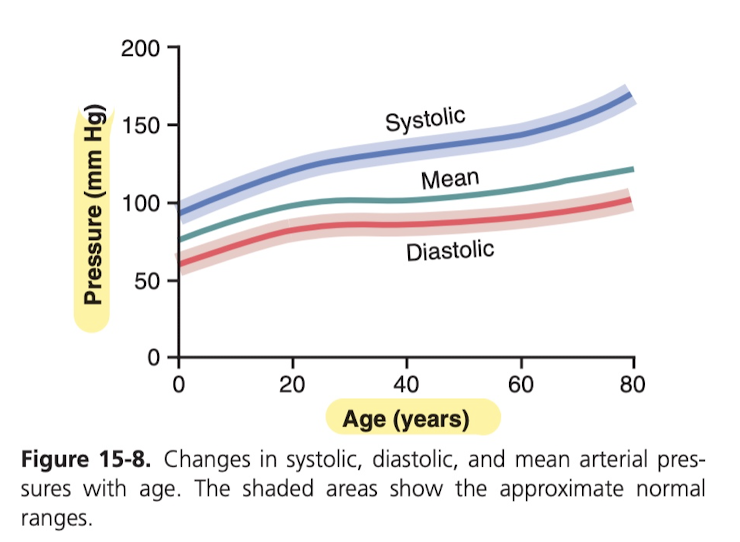
Increasing the diameter of a vessel from 1 to 2 to 4 diameter increases blood flow from 1 to 16 to 256 (4th power).
Blood is 3 times as viscous as water (it's due to the red blood cells, they drag against the wall of the blood vessel). Blood plasma on the other hand (blood but with all the red blood cells taken out) is 1.5 times that of water.
Hematocrit: volume of red blood cells to total volume of blood. Having a hematocrit of 40 means 60% is plasma. Women have slightly higher hematocrits. Varies a lot by activity, where you live, anemia, etc.
The spleen is what is responsible for cleaning the blood. It basically squeezes the red blood cells and the fragile ones break and all the released hemoglobin and other stuff is digested by the spleen and used to recreated new red blood cells. The spleen also contains cells that will remove debris, bacteria, parasites, etc from the blood, and it actually enlarges just like the lymph nodes during chronic infection to perform its function more rapidly.
It is rare that any single functional cell is more than 20 to 30 micrometers away from a capillary. That's amazing.
Capillaries are really just tiny blood vessels, so small and so thin and have such a thin layer (of endothelial cells) and they have little pores so that certain substances diffuse with ease with the interstitial spaces. Now in the brain, the junctions between the endothelial cells is extra small, called tight junctions, that only allow the tinies molecules through, like water, oxygen, and carbon dioxide. The liver has the opposites, the clefts between the endothelial cells are huge so almost everything in the plasma can pass through into the liver tissues.
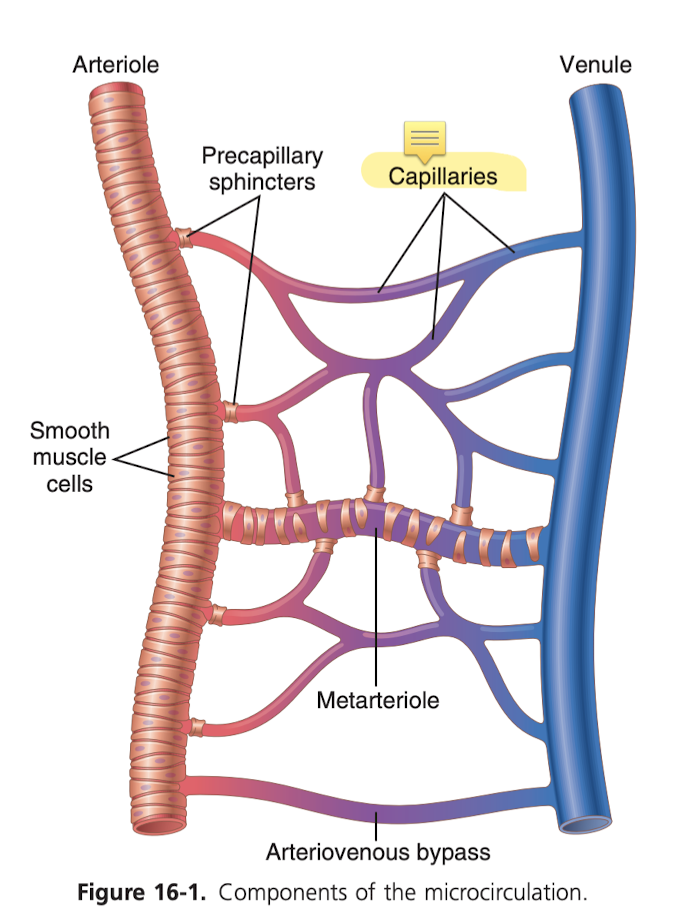
Lipid soluble substances can diffuse directly through the membrane without going through a pore, such as oxygen and carbon dioxide (generally, concentration of oxygen is higher in the blood and carbon dioxide is higher in the interstitial fluid, so oxygen diffuses into the interstitial fluid, etc.). Water soluble substances cannot pass through (water, sodium, chloride, glucose), and so they have to pass through the tiny intercellular cleft.
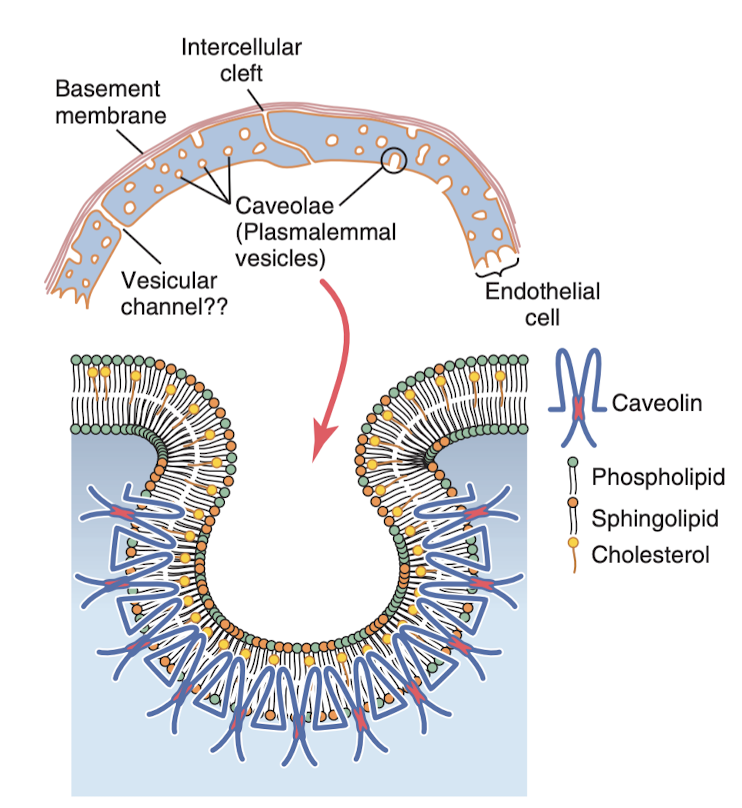
1/6th the entire volume of the body is the spaces between cells (called the interstitium, the fluid in these spaces is the interstitial fluid). These spaces contain collagen fiber bundles which are extremely strong and are what give most of the strength of the tissues, and then there's also proteoglycan filaments which combined with the fluid makes sort of like a gel, which prevents the fluid from freely flowing. The amount of free fluid in normal tissues is less than 1%, but when a tissue develops edema (swelling), these pockets of fluid expand tremendously
Subcutaneous: means underneath the skin
The lymphatic system is a "scavenger" system that removes excess fluid, protein, debris and other things from the tissue spaces. Most importantly, it carries away proteins and other large particulate matter away (which can't be removed by directly absorbing into the blood capillaries). Without this function of returning proteins to the blood, we would die within about 24 hours. Wow, I always noticed how my upper thigh/near crotch would get very hot when I was sick, now I can see that it's probably because there is a mass of lymph nodes there:
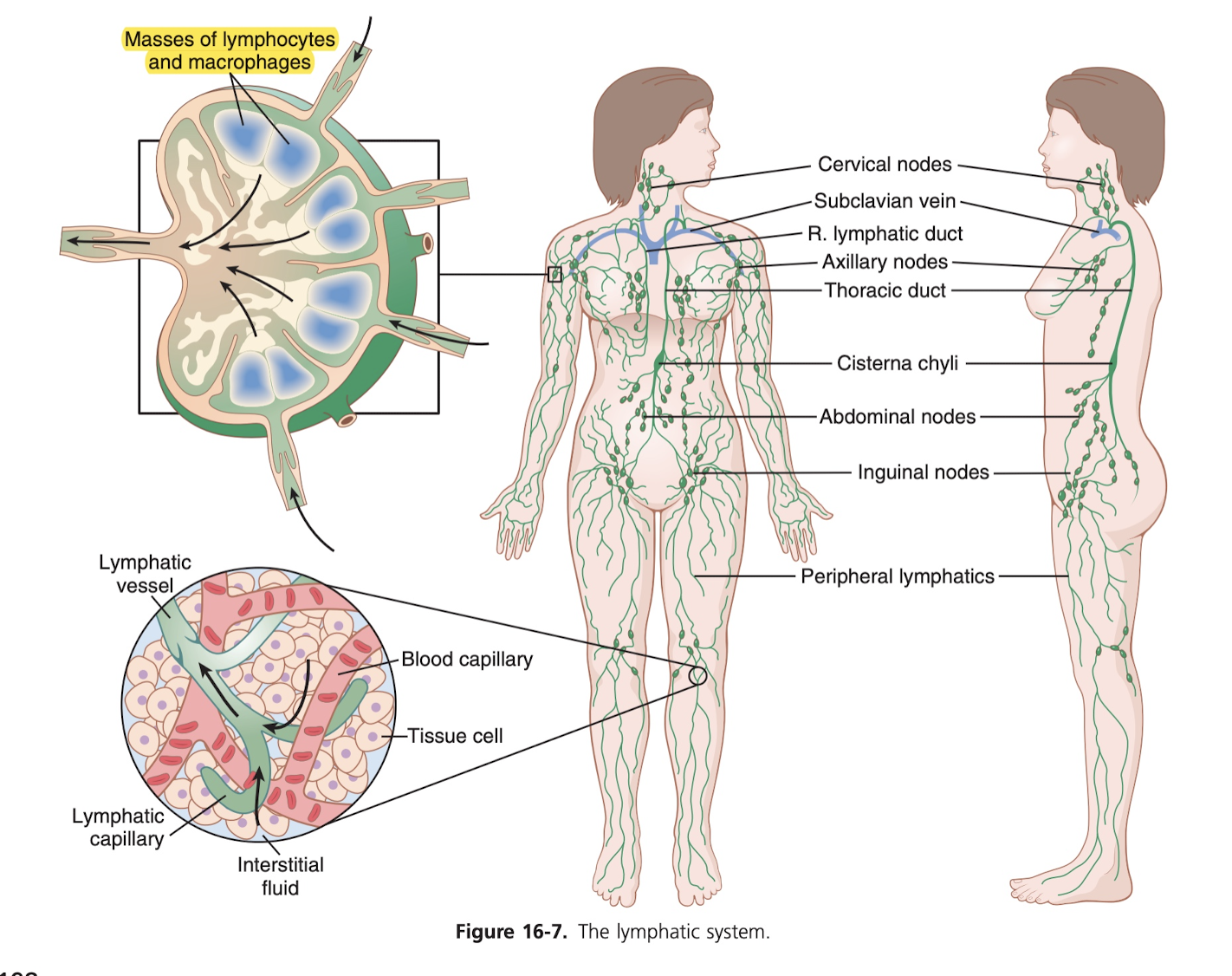
You can see that the lymphatic capillaries here are what do the reabsorption. They again are endothelial cells that kind of have little gaps/valves that they use to allow substances in. It seems like the lymphatic system is one primary way nutrients from the GI track, especially for nearly all fats from food. In fact, after a fatty meal, the thoracic duct lymph system sometimes contains as much as 1-2 percent fat. Bacteria also can get into the lymph, and as it passes through a lymph node, they are almost entirely removed and destroyed. During exercise, apparently lymph flow increases 10 to 30 fold (it's nearly 0 at periods of rest).
Thorax / thoracic (is kind of the center of the chest, between the breasts).
With regard to heat loss, the blood flow to the skin determines how much heat is lost from the body (to control body temperature). So to cool down, more blood will flow to the skin. This is fascinating and explains exactly why it's harder to run in the heat. More blood is being directed to the skin for cooling down, which means the heart is having to stroke at a higher rate/volume to get the same amount of blood to the working muscles. Blood flow is only 3ml/min/100g of tissue in cool weather, but for the entire body can be 7 to 8 entire liters per minute for the entire body, which is like twice the amount of blood you have.
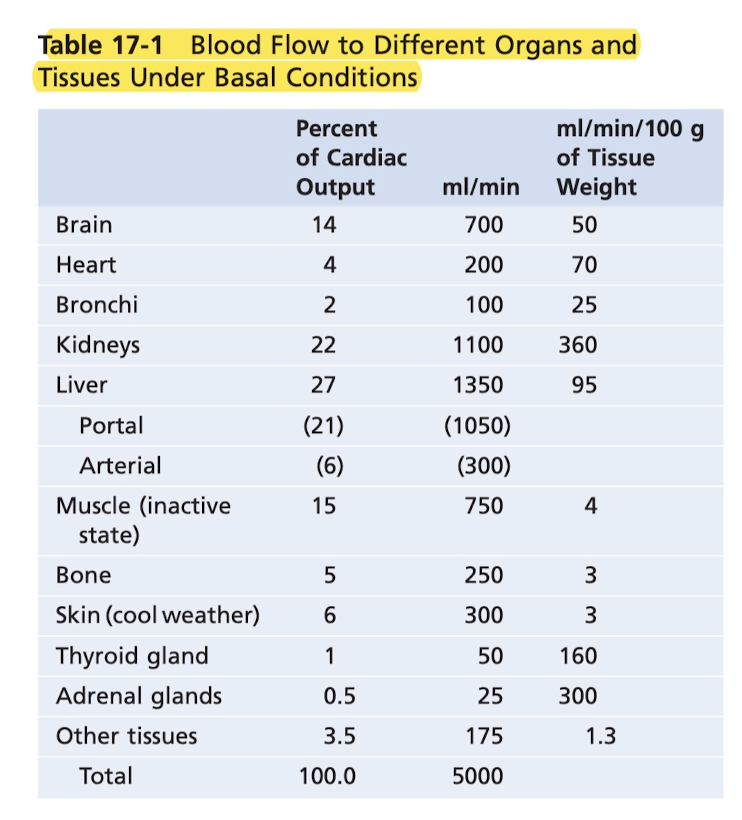
Notice how the kidneys have the highest blood flow per gram of tissue weight, due to the filtering.
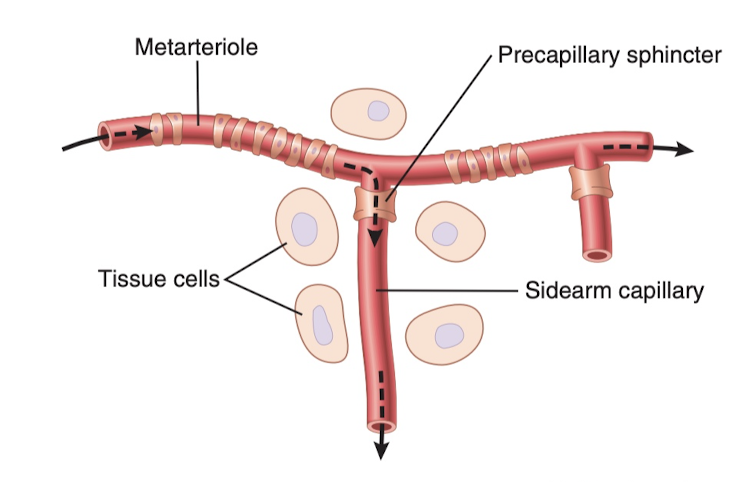
The less oxygen and other nutrients in a tissue, the more the tissue forms vasodilator substances, which are believed to diffuse through to the arterioles and cause dilation (to increase blood flow). The sphincters actually constrict to block the blood flow in a rather binary manner, opening and closing a certain number of times per minute to regulate the blood flow based on the tissue's needs. Note that the sphincters are smooth muscle, which requires oxygen to stay contracted. This makes a lot of sense, because when there's sufficient oxygen, it closes, when there's not enough, it opens, so it's self regulating.
Whenever a tissue is active (exercise, brain undergoing mental activity), the rate of blood flow increases. The increased local metabolism of the cells causes the cells to nutrients and also release vasodilator substances. This could be 20 fold increase in blood flow for skeletal muscle.
Another beautiful example of autoregulation/negative feedback: during rapid arterial pressure increases, that means more blood flow, say it's excess blood flow, well the excess oxygen and other nutrients wash away the vasodilators and the excess oxygen causes the blood velds to constrict and return flow to normal (despite the pressure).
afferent and efferent: afferent means "toward"/"close to". efferent means "away from".
Interesting, endothelial cells also release vasoconstrictor substances (e.g. endothelin). It's present in most blood vessels, but greatly increases when the vessels are injured. This helps prevent excessive bleeding in arteries that might have been torn open or by injury.
One main way local blood flow is regulated is to change the vascularity (number of blood vessels), in tissues. For instance, if metabolism is increased for a while in a tissue, vascularity increases (this process is called angiogenesis). Likewise, if it's decrease, vascularity decreases. This process happens rapidly (within days) in young animals. It happens more rapidly in new tissues, and therefore takes longer in an elderly person.
Steroid hormone have the opposite effect on small blood vessels as oxygen. It actually dissolves vascular cells. Angiostatin is a drug used on cancerous tumors to do exactly this; it prevents blood vessel growth to try to starve the tumor.
When a vessel is occluded, often collateral vessels will be formed to substitute. This usually works fine, although not during strenuous activity. By age 60, most people have experienced closure or partial closure of at least one of the smaller coronary vessels, but they aren't aware because of the collateral vessels which have prevented myocardial damage (ischemia).
Absolutely incredible: during coronary artery bypass graft procedure, the saphenous vein (big len vein) is sewn to the aorta and the coronary artery. It's obviously a vein, but after a couple months of being used as an artery, it actually changes its cellular formation to have a wall thickness similar to an artery.
Histamine is released in almost every tissue of the body if it is damaged or subject to an allergic reaction.
Autonomic nervous system contains (sympathetic nervous system, and parasympathetic nervous system). Small arteries and arterioles are connected to the sympathetic nervous system, and stimulation allows increased resistance to blood flow (and therefore decreased rate of blood flow) through the tissues. The hypothalamus plays a special role in controlling the vasoconstrictor system.
Some experience show, in anticipation of exercise, the sympathetic system might cause vasodilation of skeletal muscles to allow an anticipatory increase in blood flow even before the muscles require increased nutrients.
During heavy exercise, arterial pressure rises 30-40 percent (increases blood flow about 2x). During extreme fright, blood pressure rises sometimes 75 to 100 mm Hg within a few sections, which is to supply blood to the muscles of the body that might need to respond instantly to enable fight/flight.
Baroreceptor reflex is the best known control of artery pressure. This is managed by "baroreceptors", which are pressure receptors in the walls of several arteries. They're basically stretch receptors, and detect being stretched. Excitation of these receptors causes a response of decreasing cardiac output and peripheral resistance (vasoconstriction). Low pressure has the reverse effect, so these baroreceptors go both ways. These are actually critical for handling when you sit up after lying down. As soon as you stand up, the arterial pressure in the head falls and too big of a fall in pressure would cause loss of consciousness. But this falling pressure triggers an immediate baroreceptor reflex, causing sympathetic discharge which minimizes the decrease in the head/upper body. It also is important in reducing minute by minute variation in arterial pressure due to moving around, etc.
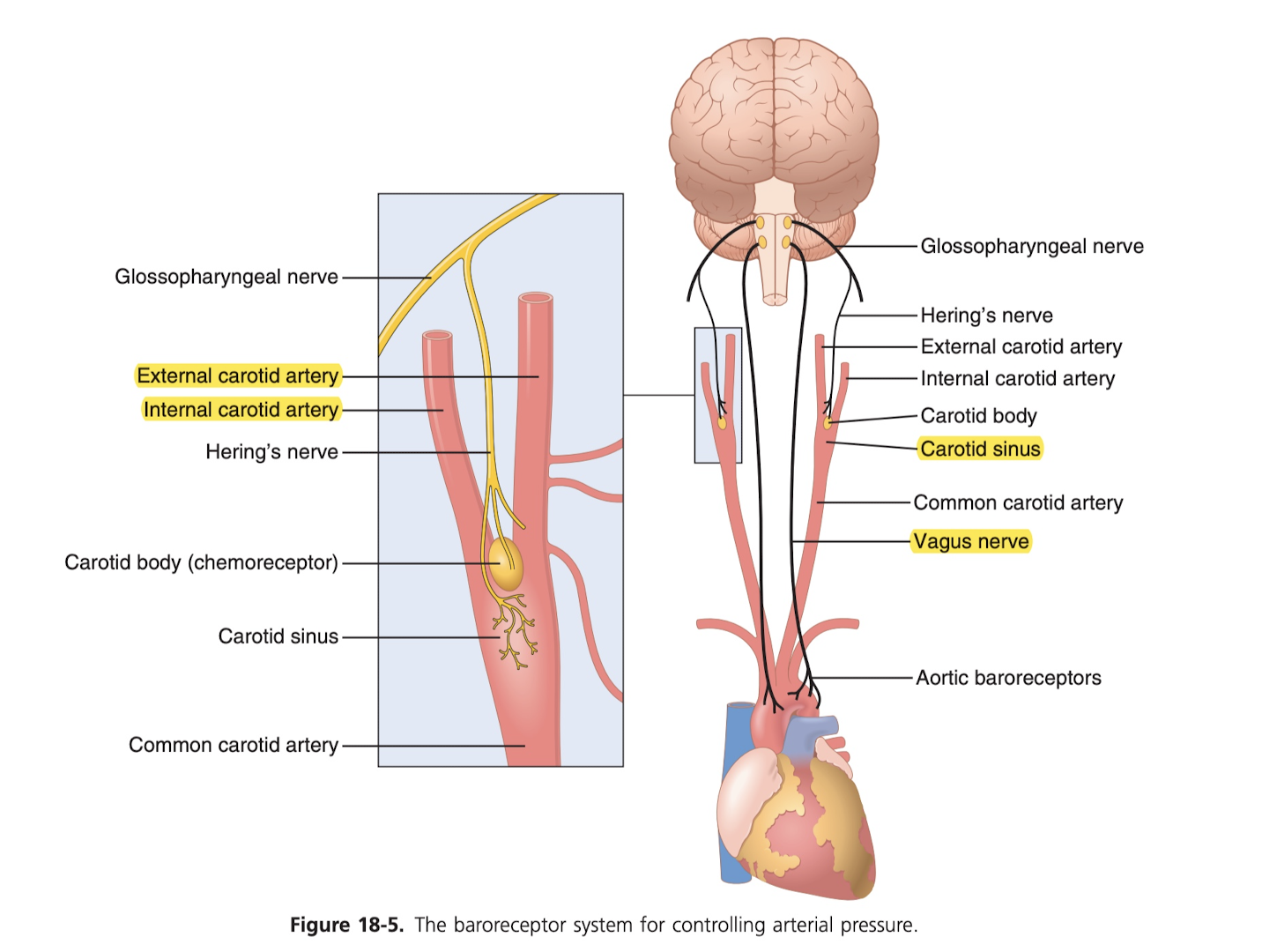
Fascinatingly, baroreceptor reflexes that detect long increase in arterial pressure may cause decreases in renal (kidney) nerve activity, which promote increased excretion of sodium and water, which in turn causes a decrease in blood volume, which helps restore arterial pressure to normal.
Similar to baroreceptors, there are chemoreceptors. These are sensitive to low oxygen, too much carbon dioxide, and excess hydrogen. When there's a decrease in blood flow, that means less oxygen and more carbon dioxide etc, and so they're stimulate, which excise the "vasomotor center" (in charge of constriction/dilation of blood vessels). The chemoreceptor reflex doesn't actually kick in though until arterial pressure is below 80 mm Hg.
Very interestingly, stretching of the atria (as in, the two heart chambers), causes reflex dilation of the afferent arterioles (close to the heart) in the kidneys (this for some reason causes "glomerular capillary pressure" to rise, will need to cover this in the kidney section, which increases filtration of fluid by the kidneys. At the same time, a signal is sent to the hypothalamus to decrease the secretion of antidiuretic hormone (ADH), which reduces the reabsorption of water from the kidney's tubules. So basically you're telling the kidney to go into overdrive and also reduce the absorption of water while you work harder.
When the lower brain stem (medulla) has sufficiently reduced blood flow (nutritional deficiency) to cause cerebral ischemia, certain neurons are strongly excited which causes arterial pressure to shoot through the roof (as high as it can). Impressively, this vasoconstriction can be so great that somme peripheral vessels become totally occluded.
The "Cushing reaction" is a special type of ischemic response that happens when the cerebrospinal fluid (the fluid the brain is suspended in) is of too high of pressure, high enough that it essentially matches the arterial pressure and starts to compress the entire brain and the arteries supplying it blood. It causes arterial pressure to rise to help maintain blood flow to the brain. This would be triggered during concussion, brain hemorrhage, tumors, etc.
When skeletal muscles contract during exercise, they compress blood vessels throughout the body. Even the anticipation of exercise tightens the muscles. This tightening translocates (redistributes) blood from the peripheral vessels to the heart and thus increases cardiac output. This is essential in causing the 5-7 fold increase in cardiac output which occurs during heavy exercise. Blood pressure can go up to 160mm Hg greater during intense exercise (usually around 100 mm Hg, though).
Fascinatingly, each respiration (breath) causes arterial pressure to rise/fall about 4 to 6 mm Hg. Couple of causes: many of the breathing signals that come from the medulla spill over to the vasomotor center with each breath, also every breath causes the thoracic cavity to become more negative in pressure, which expands the blood vessels in the chest and reduced the amount of blood returning to the heart momentarily, reducing cardiac output / arterial pressure, etc. During deep respiration, blood pressure can go up and down 20 mm Hg each breath.
The kidneys are critical in maintaining blood pressure over long periods of time. If you increase blood volume, say through infusion or drinking a lot of water, then this raising pressure directly causes the kidney to excrete the excess volume (called pressure diuresis) and salt (called pressure natriuresis) in order to restore blood pressure. At too low of a pressure, the kidneys excrete less volume than is ingested.
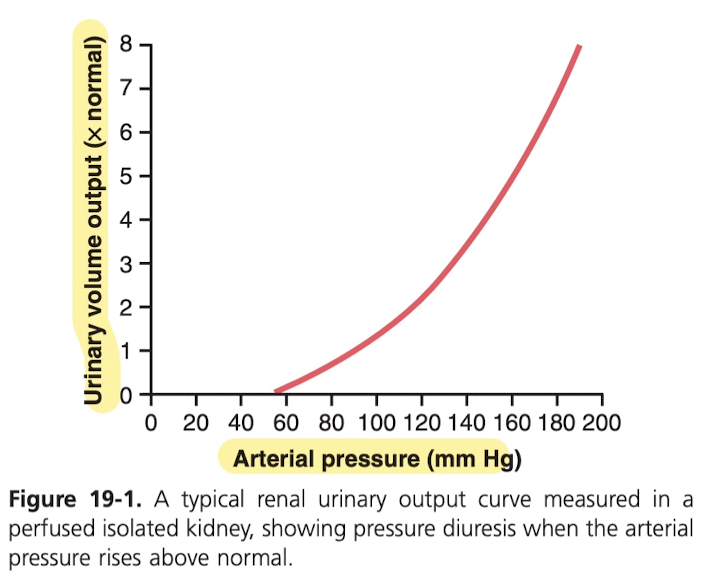
So basically this is the acute curve for an increase in blood pressure, so you'll see an increase in urinary output as the blood pressure goes up, but looking below at the chronic curve, the kidney are incredibly good at keeping the blood pressure normal even with very, very high intake (6x normal intake of water), so you can be sure that you won't increase your blood pressure long term by drinking too much water.
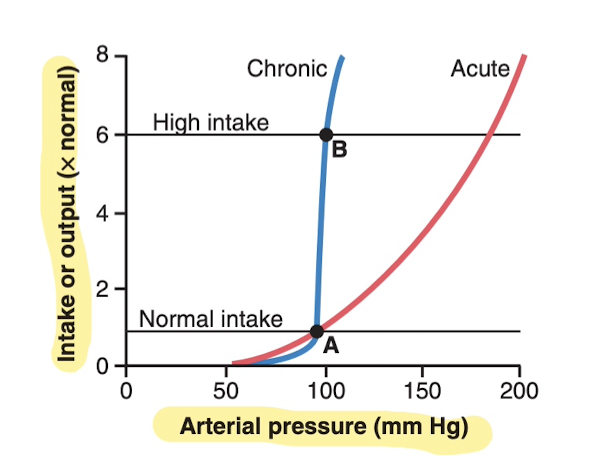
Many people are "salt insensitive", because you can have a 6x increase in salt intake and barely any change in BP, or 1/6th normal and barely any change in BP. Kidneys are just really good at regulation. Those with kidney injury though or other conditions are "salt sensitive", where even a moderate increase in salt increases BP significantly. There is also evidence that long-term high salt intake lasting for several years may actually damage the kidneys and make them more salt sensitive.
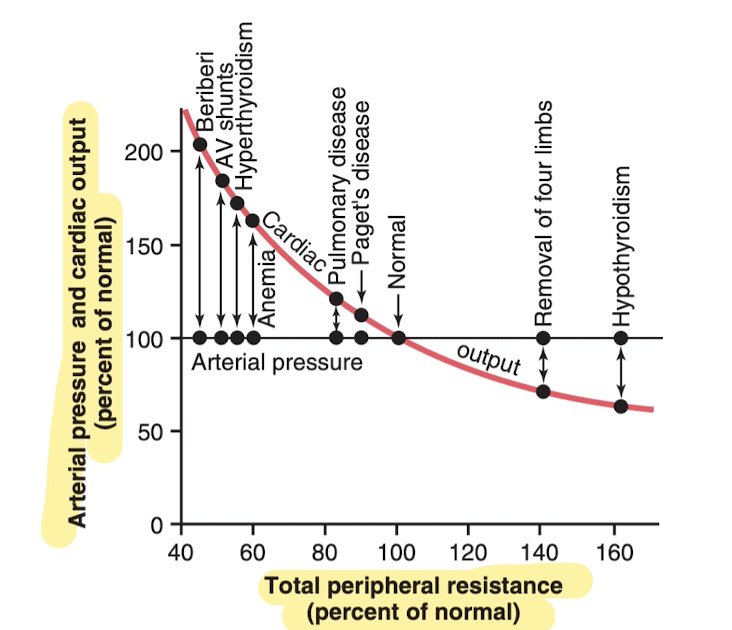
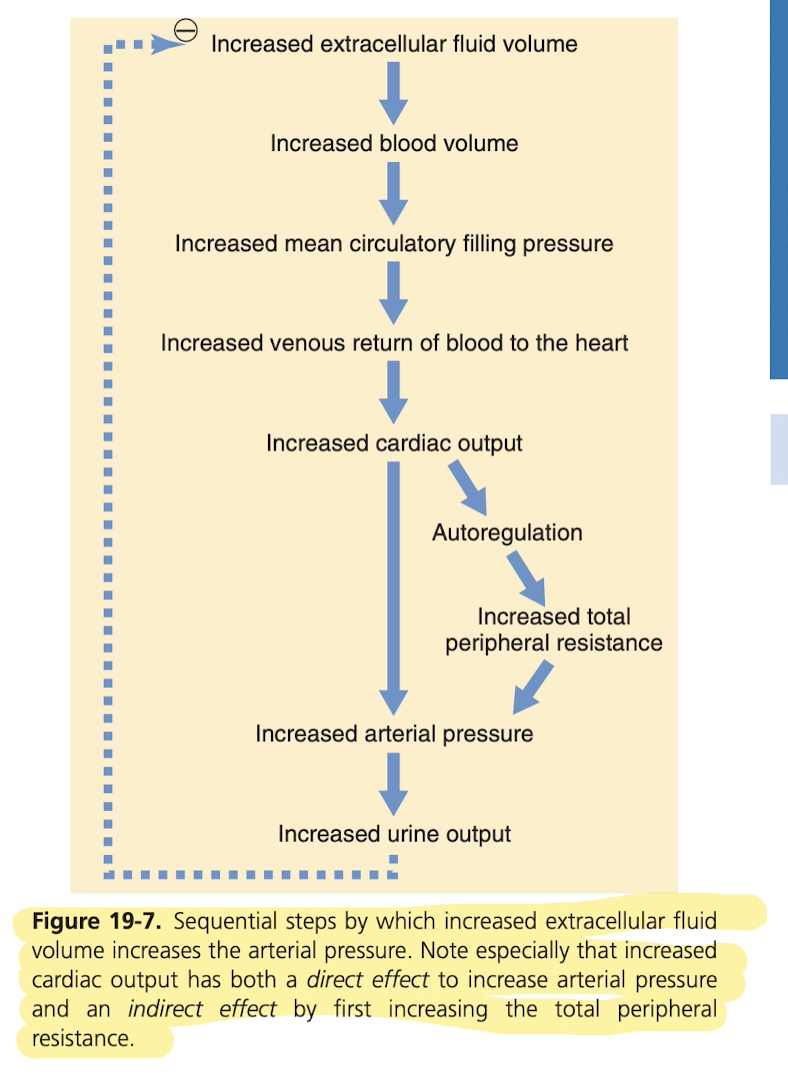
It does seem as though an increase in salt is "far more likely" to actually increase arterial pressure than an increase in water intake, because apparently pure water is excreted by the kidneys almost as rapidly as it's ingested, but that's not the state for salt. Increased salt intake then builds up in the body, and this causes extra extracellular fluid volume for two reasons: excess salt causes the thirst center of the brain to stimulate thirst, which leads to more water intake, an attempt to return the salt concentration to normal. It also stimulates the secretion of anti-diuretic hormone, which causes the kidneys to reabsorb more water from the "renal tubular fluid", which I'll need to understand what that means. So basically, that decreases the urine output which increases extracellular fluid volume to get a better ratio.
When someone has hypertension (high blood pressure), this means their mean BP is high. Above 135 mm Hg systolic (arterial pressure) is hypertensive, and above 90 mm Hg diastolic. Combined, this means the mean blood pressure is greater than about 110 mm Hg, and that counts as hypertension. Even moderate hypertension leads to shortened life expectancy, and at very high pressure (50% or more of normal), you can expect to only live a few years without treatment.
- Excess workload on the heart causes early heart failure and coronary heart disease, often causing death from heart attack.
- It also damages a major blood vessel in the brain, followed by death of major portions of the brain, cerebral infarct (called "stroke"). Depending on the region, it could cause paralysis, death, dementia, blindness, or others.
- It also almost always causes kidney damage, resulting eventually in kidney failure, uremia, and death.
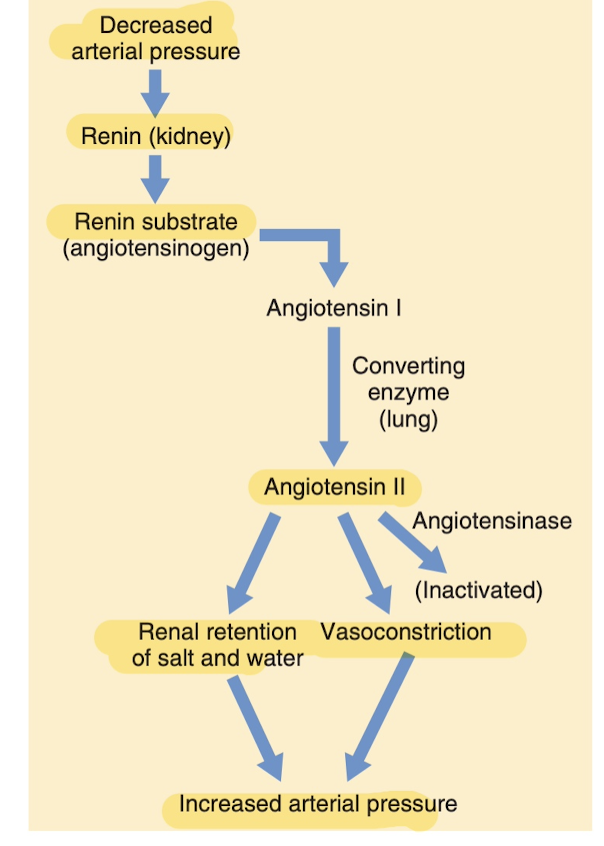
This is a key thing, the release of angiotensin causes the arterioles to constrict and the kidneys to retain more salt and water, which kicks in when there's a hemorrhage to raise BP back up to a normal level.
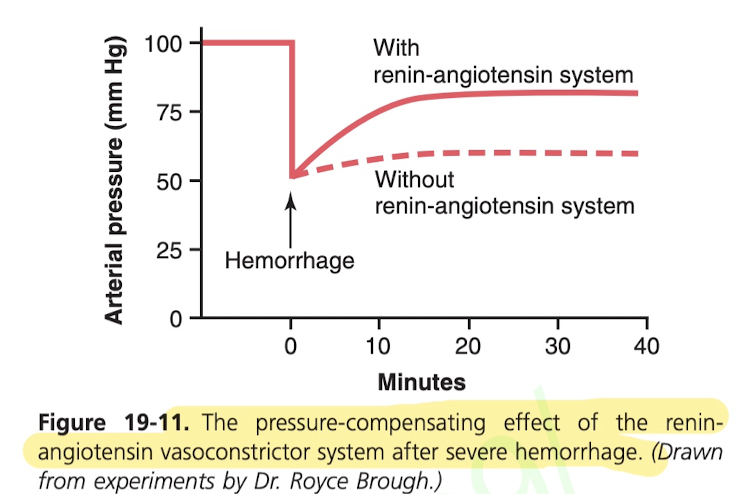
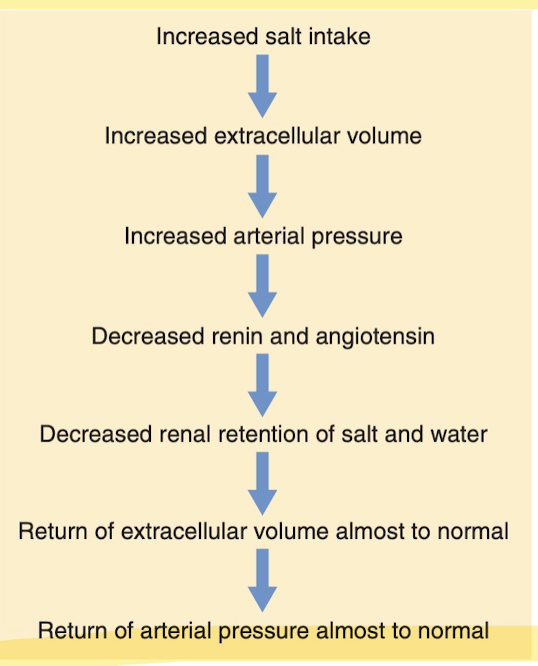
Here's another very key thing, the mechanism by which blood pressure is regulated when salt intake is increased greatly. The kidneys just have this mechanism built in to regulate when there's an increase in arterial pressure. When this is working normally, it's incredible: pressure rises no more than 4-6 mm Hg in response to a 100 fold increase in salt.
The kidneys can kind of fuck each other over. So if there's stenosis (narrowing) of one artery of one kidney from something like atherosclerosis, it will decrease renin and angiotensin release because it thinks BP needs to be increase, but in fact it doesn't, but that causes the other kidney to also retain salt and water, and that causes BP to increase extra.
Acute neurogenic hypertension can be caused by strong stimulation of the sympathetic nervous system (such as when someone is very excited or experiencing anxiety), and peripheral vasoconstriction occurs everywhere and acute hypertension occurs.
Excess weight gain also leads to stimulation of the sympathetic nervous system to causes chronic hypertension. This is known as "primary/essential hypertension", which is essentially when the cause is unknown, but appears to be due to a sedentary lifestyle and weight gain (65% to 75% of the risk). Likely causes
- Increase cardiac output due to extra adipose (fat) tissue, and blood flow to all the other organs also increases because they have an increased metabolic need.
- It's possible that the hormone leptin is released from fat cells which may directly stimulate the hypothalamus which in turn stimulates sympathetic activity (causing increased BP). This isn't fully understood.
Often treated with:
- Weight loss if possible
- Otherwise, vasodilator drugs (increase blood flow to kidneys, but also everything else because they're not specific enough)
- Natriuetic or dietetic drug that decrease reabsorption of salt and water.
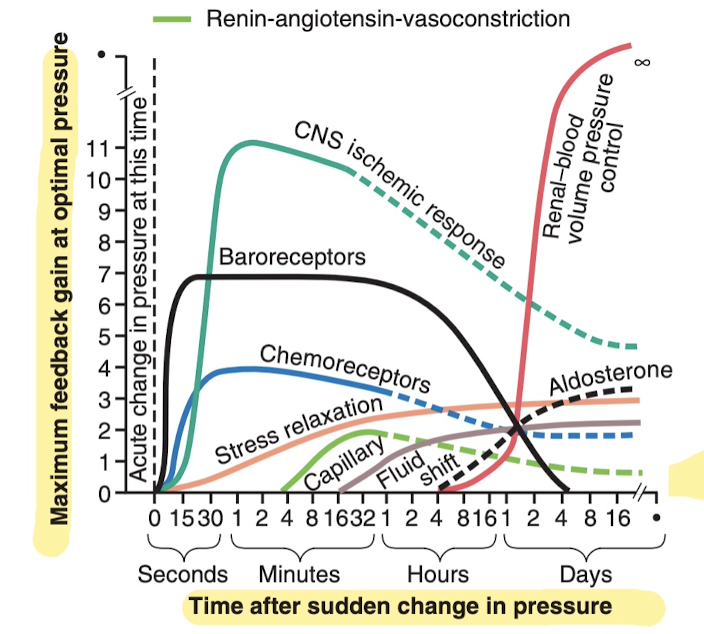
Blood pressures salt sensitivity varies person to person, and changes as you get older (gets more sensitive).
After severe hemorrhage that causes acute drop in blood pressure:
- Constriction of the veins to transfer blood to the heart.
- Increased heart rate and contractility to increase pumping capacity
- Constriction of the peripheral arterioles to impede blood flow out of the arteries (would like to learn more about this but not much online).
Cardiac output is the quantity of blood pumped into the aorta each minute.
- Dependent on level of body metabolism
- Whether person exercises
- Age
- Size
- (young men at rest, 5.6 L/min, women is 4.9 L/min).
- Rises rapidly to 4L/min by age 10 and declines to 2.4L/min by age 80.
Venous return is the quantity of blood flowing from the veins into the right atrium each minute.
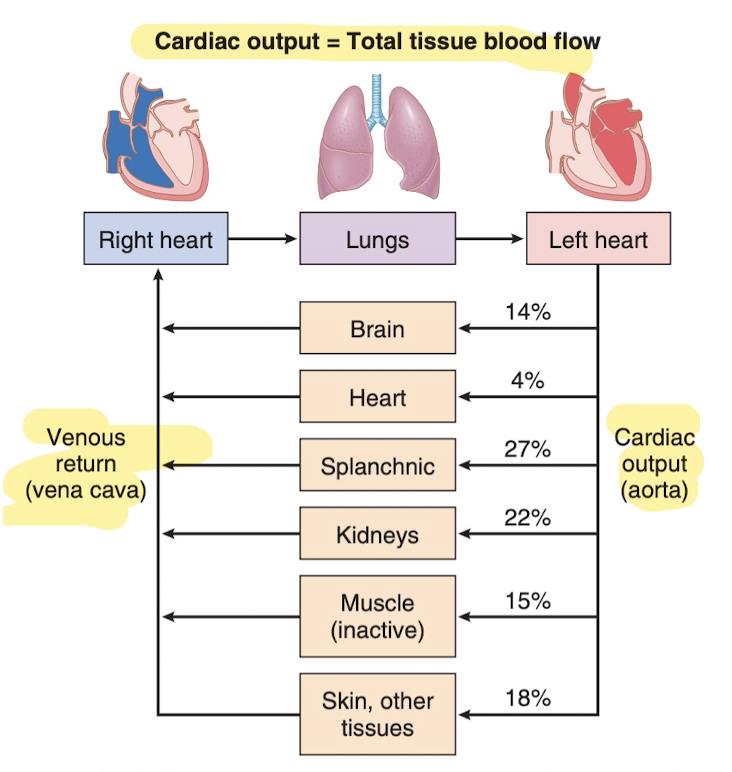
Through exercise:
- Marathon runners increase heart massy by 50-75%.
- Heart can pump as much as 30-40L/min, 2.5 times the average person.
- This increased pumping is one of the most important factors in determining running time.
During exercise:
- Increased metabolism of skeletal muscles acts on muscles arterioles to relax them (dilation) to allow more oxygen/nutrients to flow.
- The brain simultaneously sends a signal to the veins to constrict, increase heart rate, etc. These causes more blood to flow through the muscles.
- BP actually increases and is responsible for an extra 30 to 100% increase in cardiac output.
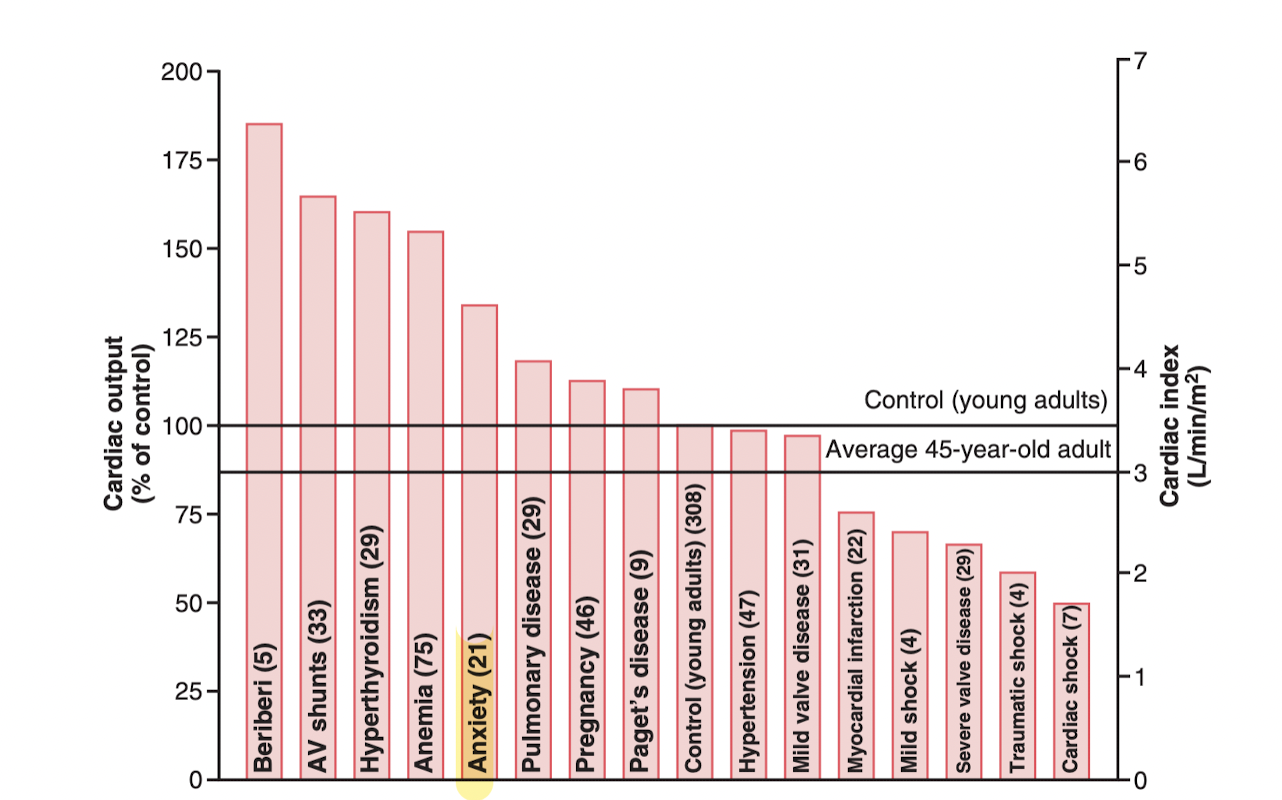
Remember that cardiac output is stroke volume times stroke rate. So just how much blood is being pumped out of the heart per second. Anxiety is pretty high up there, but Anemia beats it out (makes sense, less hemoglobin means less oxygen per liter of blood, so more blood needed). Apparently the blood is less viscous too, which is another contributer.
Hyperthyroidism is the increase in metabolism of most body tissue, which means more oxygen needed.
Another decrease in cardiac output is reduced blood volume (severe hemorrhage aka bleeding out). Not enough blood in the peripheral vessels for them to constrict enough to create pressures high enough to pump blood back to the heart.
Intrapleural pressure is the pressure in the chest cavity.
Cardiac output can actually be measures with echocardiography, which presumably uses sound waves to measure the size of the heart cavities and even the velocity of the blood flowing from the left ventricle into the aorta.
During muscle contraction, the blood flow to the muscle actually slows down due the compression of blood vessels. During strong tetanic contractions, blood flow can actually stop entirely, causing rapid weakening of the contraction.
During muscle activity, oxygen is used rapidly obviously, which makes the arterioles less able to maintain constriction due to lack of oxygen, which causes them to dilate, which of course allows more oxygen to flow. That's a nicely direct method of compensation.
In the onset of exercise (sympathetic activation), the heart greatly increases heart rate and pumping strength. Then the arterioles of peripheral circulation are strongly contracted (except in the active muscles, which are vasodilated). Basically, the nonactive muscles and nonvascular areas of the body are temporarily reducing blood flow to "lend" to the active muscles. The two peripheral systems spared are the coronary and cerebral system, since the brain and heart obviously are needed in exercise.
Blood pressure goes up during exercise, between 20 mm Hg and 80 mm Hg, but actually when doing full body, like running or swimming, it's only 20 to 40, because there's vasodilation in such a wide number of muscles.
When it comes to running at least, having a high cardiac output is just as important as having strong muscles. Generally the record breakers are the ones who have the largest cardiac output.
One third of deaths in industrialized countries in the western world are from coronary artery disease, and almost all old people have at least some impairment in coronary artery circulation.
Very interesting: when the cardiac muscle is ischemic or aerobic (cut off from oxygen supply specifically), it relies on aerobic glycolysis, which of course produces lactic acid, which is probably the cause of the cardiac pain/burning in cardiac ischemic conditions.
You only have 30 minutes of coronary ischemia before injury and death of cardiac cells starts to occur.
Frequent cause of diminished coronary blood flow is atherosclerosis. In people overweight, have sedentary lifestyle, or have high blood pressure and damage to the endothelial cells of the coronary blood vessels, cholesterol begins to become deposited beneath the endothelium, and eventually become calcified, and protrude and block blood flow. Common place for this to start happening is the major coronary arteries.
Acute occlusion of the coronary artery is usually in people with existing atherosclerosis. This is the really scary part. The plaque has broken through the endothelium, and coming directly in contact with the flowing blood. Since the plaque is unsmooth, platelets adhere to it and clot, and red blood cells all build up with it and it creates are hard clot, which blocks the vessel. Occasionally it breaks away and flows to another branch of the artery and blocks it there. When that happens it's called a coronary embolus.
After a coronary occlusion, the other smaller (collateral) coronary arteries dilate within seconds. This usually provides less than 1/2 that needed to keep alive most cardiac and does not increase much for 8-24 hours. Then by second or third day it's doubled, often making up all or almost all the blood flow within 1 month. Due to this, patients recover almost completely from coronary occlusion when area of muscle is small enough.
When atherosclerosis constricts arteries over years, collateral vessels begin to form as it gets worse, so you may not get an acute cardiac issue. Eventually, it gets so bad even the collateral vessels can make up for it, or they too develop atherosclerosis. Heart muscle begins to suffer, and can't cannot pump normal amounts of blood. This is one of the most common causes of cardiac failure in older people.
Within a few hours of almost no blood supply, the cardiac muscle cells die.
Systolic stretch is horrifying: basically when you have damage to the heart, say from some coronary occlusion from before, and it results in dead (ischemic) muscle, then each time the heart beats (depending on its location) it stretches out instead of contracting. Which for one reduces the pumping force of the heart of course, but for two the ischemic portion begins to die, and the first day or so is okay, but a few days later it becomes thinner and thinner, and bulges more, and the heart then ruptures and all of the blood fills into the pericardial space and causes cardiac tamponade, where the sack is filled and prevents the heart from pumping, and the patient dies almost instantly.
When the heart is not able to pump with sufficient force, and there starts to be cell death in cardiac muscle and other tissues, this is call coronary/cardiac shock. This occurs when more than 40% of the left ventricle is infected. 70% of people die once cardiac shock develops.
When the heart is struggling, that means blood is damming in various areas, which increases capillary pressures everywhere, but especially the lungs. This is usually fine for the first few hours. But after a few days, the reduced output means less blood to kidneys, which means less urine to reduce blood volume. Too much builds up, and after a few days of seeming fine, will suddenly have acute pulmonary edema (too much fluid) and they'll die within a few hours of symptoms.
Coronary occlusion can also cause sudden ventricular fibrillation. Especially likely after a large infarction. It's especially likely in the first 10 minutes after the infarction, then safety for a while, then 1 hour to a few hours it's likely to occur. Also possible days after but less likely.
The heart is able to recover either partially or almost completely within a few months, from cell death of heart tissue (which becomes scar tissue, rest of the heart hypertrophies to make up for the loss).
There is high value in resting during myocardial infarction. You need maximum blood throwing through the collateral channels, and if you're exercising or stressed or anything that elevates workload on the heart, it diverts blood from these collateral channels to the main ones, which worsens the ischemia. This is called "coronary steal" syndrome. So one of the most important things here is absolute body rest during recovery.
Although a person can't "feel" their heart, ischemic heart muscle does cause a pain sensation. It's not known what causes this. It's thought maybe the ischemia causes the muscle to release acidic substances, like lattice acid, that aren't removed fast enough by the slow blood flow.
Angina Pectoris (cardiac pain), which is the pain felt during a heart attack. This is when load on heart exceeds coronary blood flow. Felt upper sternum over the heart, and referred pain commonly on the left arm and left shoulder but also frequently in the neck and even side of the face. "The reason for this distribution of pain is that during embryonic life the heart originates in the neck, as do the arms. Therefore, both the heart and these surface areas of the body receive pain nerve fibers from the same spinal cord segments."
Most people have angina pectoris when they exercise or are emotional. It's worse in cold temperatures and having a full stomach (which increase workload of the heart). Usually only lasts a few minutes. Described as "hot, pressing, and constructing", and makes you want to cease all body activity.
Nitroglycerin is a vasodilator (and other nitrate drugs).
Coronary artery angioplasty, since the 1980s this has been used to open partially blocked coronary vessels before they become totally occluded. A small balloon tipped catheter is passed (while using x-rays to watch it) into the coronary system until it gets to the area that's blocked (say by a bunch of built up plaque, from atherosclerosis or a thrombus), and then the balloon is inflated which really stretches the artery, then removed, which opens vessel for flow. Usually increased 3 to 4 foot and more than 75% of patients are relieved of coronary ischemic symptoms for at least several years. Although they often still require coronary bypass surgery. Sometimes stainless steel mesh tubes (stents) are placed around the balloon and left in place to hold the artery open. Within a few weeks of it being placed, the endothelium grows over the metal to allow blood to flow smoothly over it. Unfortunately, reclosure of the artery occurs in 25 - 40% of patients treated with angioplasty, often with in 6 months.
New procedures are being used to open coronary arteries. One of them uses a laser beam to dissolve the atherosclerotic region without damaging the rest of the artery.
Definitions and other facts
Renal means of or relating to the kidneys because renal used to mean kidney in french.
Vascular capacitance refers to the ability of a blood vessels ability to expand to accommodate more blood volume without a sizable increase in blood pressure. So I would guess veins for instance have a high capacitance because they can expand to hold a large amount of blood.
When more volume is added to the blood without an increase in vascular capacitance (expanding of blood vessels), then obviously there's an increase in blood pressure.
Vena cava: the large vein carrying blood back to the heart. There are two branches, the superior (above) vena cava, which returns blood from the brain and the inferior (below) vena cava which returns blood from the body.
Stenosis: means a narrowing.
Anuria: failure of the kidneys to produce urine.
Epi- means on top of, upon, above.
Endo- means inside, within, internal.
-itis - means inflammation.
Exsanguination as a method of slaughtering animals makes more sense now: you incapacitate them (knock unconscious) with a bolt gun, then sever their carotid arteries and jugular vein (the vein that returns blood from the brain to the heart). The reason you flip them upside down is simple: there's more blood in the body than there is in the head/neck, and so you're just letting that blood pool in the heart, then get pumped out by the heart through the aorta. If you didn't invert, then the heart might only be able to pump out the smaller quantity of blood above it (head/neck), because it would start to get weaker due to the blood loss and would be fighting partially against gravity at that point.
Blood is always red. Oxygenated blood is bright red, and deoxygenated blood is dark red. The reasons veins appear blue is the scattering of light by the skin at different wavelengths combined with the fact that it's dark red.
The clavicle is slightly bent S-shaped bone (collarbone) that you can see in most people below their neck that stretches between their shoulders. Subclavian vein refers to the vein that tucks just underneath this bone.
Brachial means relating to the arm. The brachial vein is one of the many veins in the arm.
Skimmed through part of chapter 20, it's just too in depth on the cardiac output/venous return curves.
Fun facts
- Search the document for “interesting”, “fascinating”.
- There are 100 trillion cells in the human body. 25 trillion are just red blood cells. Even in adult humans, billions of cells die every hour in tissues like the intestine, bone marrow, and are replaced by new ones.
- Tetanus has nothing to do with rusty nails. It's just bacteria that exists in nearly all soil that causes tetanus, it gets in through cuts.
- Skin makes up 12 to 15% of body weight.
- If you lose ~2 liters of blood (depends on height, gender), you will die without an infusion. This is called exsanguination (bleeding out).
- The whiplike motion of the cilia on the surface of the cells in the nasal passages (as many as 200 on a cell, each about 2-4 micrometers) actually moves the mucus at a rate of 1cm / minute toward the pharynx for destruction. Each celia whips 10 to 20 times per second.
- One can expect as many as 10 or (many) more mutations in the passage of genome from parent to child.
- The reason you feel pain in your left arm, left shoulder, neck, side of face, during heart attack is because during embryonic life the heart originated in the neck (same with the arms), and therefore both the heart and these areas of the body are innervated by nerves coming from the same spinal cord segments.
- For some young animals, 7/8 of the liver can be removed surgically, but because these cells are basically built to recreate themselves (undergo mitosis), it can return basically entirely to normal. This is the case for most other tissue, like the intestine
- The total amount of water that diffuses (in and out) of a red blood cell during each second is 100 times the volume of the red blood cell itself.
- Speed of transmission in nerve fibers can vary from 0.25m/s to 100m/s (more than football field length in 1 second)
- The energy required to digest each macronutrient (its DIT) can be expressed as a percentage of the energy provided by this macronutrient: Fat provides 9 calories per gram, and its DIT is 0–3%. Carbohydrate provides 4 calories per gram, and its DIT is 5–10%. Protein provides 4 calories per gram, and its DIT is 20–30%.
- "Piloeroctor muscle", causes piloerection. Each hair has a piloerection muscle which allows the hair to stand upright, also known as goose bumps.
- The sounds you hear when you listen to the heart aren’t the beating, they’re the flaps (valves) closing to enable the pressure buildup that allows the blood to be pushed out of the heart. These flaps generate vibrations that make the sounds you hear. The sounds are called S1 and S2. They should be a “lub” and a “dub”. The lub is the closing of the valves that connect the atria and the ventricles, and the dub is the closing of the valves between the ventricles and the pulmonary artery (where blood flows to the lungs to get oxygenated) and the Aorta (artery) where oxygenated blood flows to the rest of the body for use. The dub is louder because the semilunar valves are much much thicker and close faster than the AV valves. The lub is low pitch, the dub is high pitch.
- For every 1 degree F increase in body temperature, the heart rate increases by about 10 beats per minute. The increased temperature makes the sinus node more "leaky" to ions that slowly raise it back up to hit action potential, and the cycle repeats.
Practical tips
- CPR: Remember it's CAB not ABC now. 30 compressions to the beat of "stayin' alive" the song (100bpm), then head tilt and chin up to open airway. 125 pounds of force (1.5-2 inches compression); most people don't push hard enough. Pinch nose, two rescue breaths. Back to 30 compressions, etc. If they have a pulse but not breathing, just do rescue breaths.
- Choking: tip them forward slightly (so it comes out, not down). Then 5 back blows (hit between shoulder blades with heel of hand, put other arm around them to catch them), then 5 abdominal thrusts (Heimlich, above belly button, find+palm, inward and upward). Alternate back and forth. If on ground choking, try doing the same thing directly down into their belly.
- Fainting: check for CAB first, then simply have them on their back, loosen any tight clothing, and raise their legs by ~12 inches. Don't let them stand up after they regain consciousness. Cause often is vasovagal syncope (sin-cup-ee), which is just overreactive parasympathetic response (vagus nerve), reduced cardiac output (stroke volume + stroke rate) + excessive vasodilation causing blood to pool in legs and not get to heart (massive fall in blood pressure). Often triggered by: strain, stress, long periods of standing, heat, the sight of blood. Should get heart checked afterward just in case.
- Heart attack (myocardial infarction due to coronary occlusion): the person should lie down and be at complete rest to minimize the load on the heart, which will reduce cell death in the ischemic areas of the heart (the parts not getting oxygenated blood).
- Legally, if you break a rib or something while trying to help, you can't be sued.
- 5-8 minutes without oxygen, brain starts suffering permanent damage.
- Without CPR, survival rate goes down 10% every single minute.
Other good resources, small or large
- How kidneys work, laymen: https://www.nhs.uk/Livewell/Kidneyhealth/Documents/kidney%20guide.pdf
- Animation of inside cell, really stuck with me in high school: https://www.youtube.com/watch?v=B_zD3NxSsD8
- The Vestibular system: this is basically your body's built in accelerometer and gyroscope: https://www.khanacademy.org/test-prep/mcat/processing-the-environment/sensory-perception/v/the-vestibular-system-balance-and-dizziness
- It's insanely cool. Basically this is the "inner ear" stuff. This is separate from proprioception, which is the relative positioning of the limbs.
- There are these three spirals, called canals. These three canals are all orthogonal to each other, so they each are in one plane. Each canal is filled with fluid and when we rotate it causes the fluid to shift along that canal, and we can sense how quickly and how much fluid is moving, which gives rotational acceleration along that plane. This is essentially a gyroscope.
- If you stop spinning, the liquid keeps going, and the brain mismatch with this liquid causes dizziness and motion sickness. So spinning in the opposite direction actually does help slow the liquid.
- We also have another part which is just a bunch of little crystals/rocks that are attached to hair cells in this kind of gel environment (they are heavier than the gel) and when they move they actually physical pull on the hair cells, and we translate that to standing upright, lying down, or accelerating.
- Busting myths about metabolism: https://www.unm.edu/
- ~lkravitz/Article%20folder/metabolismcontroversy.html and calorie expenditure: https://www.unm.edu/~lkravitz/Article%20folder/caloricexp.html
- https://docs.google.com/spreadsheets/d/1mHlS5RPz1_Lp8KHYwElSetT2WTAbgzvyi1YV6_e3E5I/edit#gid=0
- Khan Academy has a fantastic MCAT test prep with really great videos. May want to follow this as well.
Crazy thoughts/questions
- If you wanted to manufacture something that was cancer resistant, you'd basically just want DNA that was "read-only". The best way would just be to imbed a tiny device into every cell, that's capable of itself replicating (the hard part) during mitosis. This device could create RNA just like DNA does, and all that, and everything would work fine. Another solution from computers is a check-bit, just like RAID storage. Not sure how that would work exactly.
- We will probably solve aging and cancer around the same time.
- What would it take for there to be a steady enough supply of oxygen and elimination of CO2 in the byproducts of aerobic respiration such that we could be generating 32 ATP as rapidly as we produce the 2 ATP from glycolysis? That seems to be the limiting factor.
- How can we evaluate the strain we're putting on the body? It is so good at maintaining homeostasis, that doubling salt intake barely affects BP at all, especially at a young age, so we can't use BP as a proxy for instance. What do we measure then? Probably intake I would guess, that's what matters the most, but what does that translate to. Maybe it'd be better to measure the output, like feces/urine content, because what's not used has to be filtered and that can be a strain I imagine on the kidneys etc. I just want to know how we can measure the strain, so I can know how bad my diet really is for my body, and looking at blood levels of things is an indicator for how good your body is at maintaining homeostasis right now, not how much strain you're putting on it. By the time that starts to fall, it's already too late, you have atherosclerosis or whatever. Treat be body like any other system (input, output, etc) and that's all you need to know.
TODOs
- Read through notes and bold the major terms.
- Read a pathology book: Harrison's principles of internal medicine.
- Maybe read a pharmacology book / review Camilo's wiki article he sent: https://en.m.wikipedia.org/wiki/WHO_Model_List_of_Essential_Medicines
Typos found
Figure 16-2
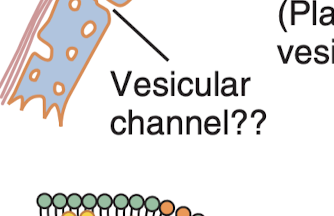
Page 232
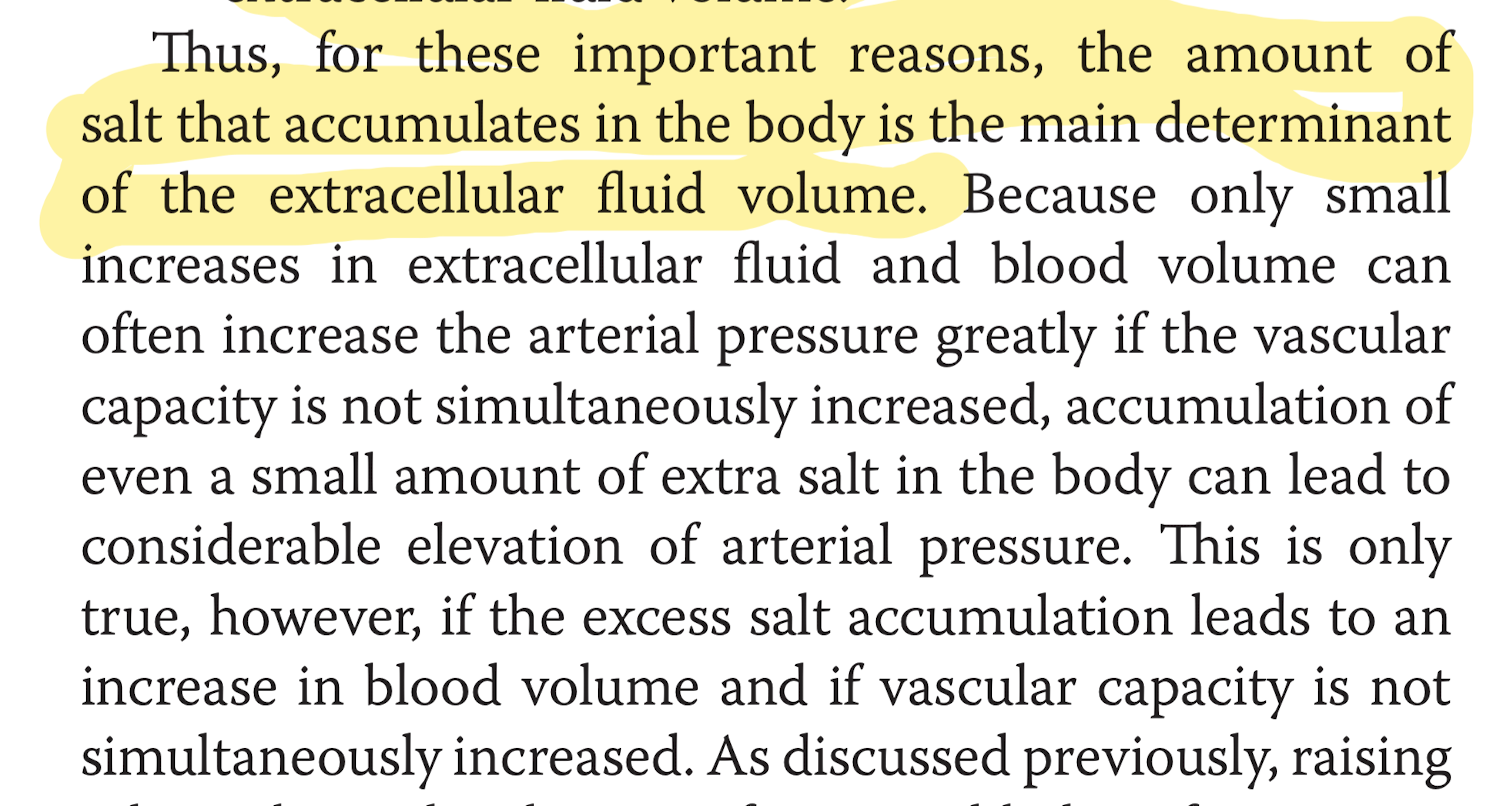

Page 274
